3
Plutonium-239
Making the plutonium isotope of mass 239 (Pu-239) was a principal objective of nuclear materials production in the United States from the 1940s through the late 1980s.1 Approximately 100 metric tons of Pu-239 were obtained from the nuclear reactors and separations facilities at the Hanford, Washington and the Savannah River, South Carolina sites for use in nuclear weapons (see Chapter 2 and Appendix A). About half of this production has been declared as surplus.2 The surplus inventory includes clean metal, mainly from disassembly of weapons; oxide; and plutonium combined with a variety of other materials in reactor fuels, targets, and miscellaneous forms (see Figure 3.1). From its fact finding, the committee believes that managing and disposing of Pu-239 is the Department of Energy’s (DOE’s) greatest nuclear materials challenge, and presents the greatest needs and opportunities for near- and longer-term research by the Environmental Management Science Program (EMSP) and other DOE programs.3
DOE’s efforts to manage surplus Pu-239 have been guided by the recommendations of a 1994 National Academy of Sciences study of plutonium disposition. The study made four specific recommendations (NAS, 1994, p. 2):
-
minimize the time during which the plutonium is stored in forms readily usable for nuclear weapons;
1 |
Plutonium is a metallic, chemically reactive element. It does not occur naturally on Earth, but it is produced in nuclear reactions. Tritium, another product of nuclear materials production, is not discussed in this report. |
2 |
According to publicly available information DOE manages approximately 99.5 metric tons (MT) of plutonium, mostly Pu-239, at eight sites. Of this inventory, 52.5 MT are excess to national security needs (DOE, 2000c). |
3 |
Other plutonium isotopes in the DOE inventory are addressed in Chapter 7 of this report. |
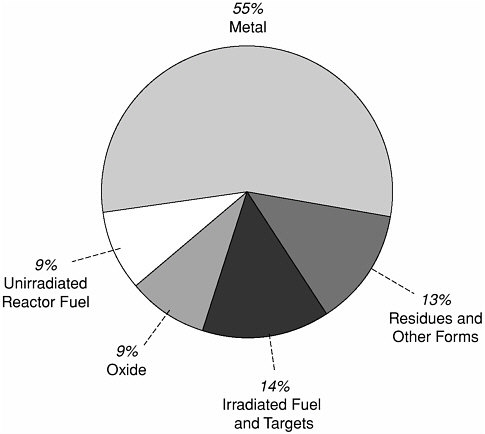
Figure 3.1 DOE has declared that about 50 metric tons of Pu-239 are in excess of national needs. About half is clean metal from disassembly of nuclear weapons and other sources. A variety of chemical and physical forms comprise the remainder. DOE plans to convert most of the excess Pu-239 to mixed oxide fuel for commercial power reactors. Source:DOE 2000c.
-
preserve material safeguards and security during the disposition process, seeking to maintain the same high standards of security and accounting applied to stored nuclear weapons;
-
[provide] a form from which the plutonium would be as difficult to recover for weapons use as the larger and growing quantity of plutonium in commercial spent fuel; and
-
meet high standards of protection of public and worker health and for the environment.
Currently efforts at most DOE sites are directed at gathering, processing, and storing excess plutonium according to the DOE 3013-2000 Standard “Stabilization, Packaging, and Storage of Plutonium-Bearing Materials” (DOE, 2000b). The standard provides for storage of stabilized, solid material in specially designed cans for up to 50 years (see Figure 3.2). Some sites, such as Rocky Flats, are being “deinventoried” of their plutonium, which is being shipped to consolidate the inventory at a limited number of sites, such as Savannah River.
A key element in DOE’s strategy for eventual disposal of its surplus inventory is the conversion of as much of the excess Pu-239 as is technically and economically feasible into mixed oxide (MOX) fuel that can
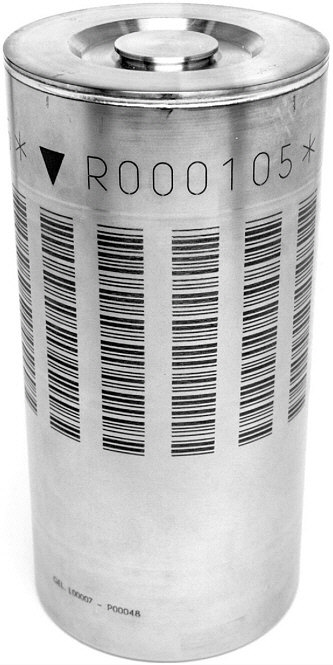
Figure 3.2 DOE 3013 cans are intended to provide safe storage of excess plutonium for up to 50 years. Use of these containers will help DOE to “de-inventory” many of its facilities, close some sites such as Rocky Flats, and consolidate the plutonium at a few locations. The 3013 cans are typically 5 to 6 inches in diameter and 10 to 12 inches long. Source: DOE Los Alamos National Laboratory.
be used to generate electricity at commercial nuclear reactors.4 The spent MOX fuel would be disposed with other DOE and commercial spent fuels, for example, in the planned Yucca Mountain repository. Most of the surplus plutonium is in the form of pits,5 clean metal, and oxides for which conversion to MOX fuel should be straightforward, based on French experience (Johnson and Brabazon, 2002). A pit disas-
sembly and conversion facility and a MOX fuel fabrication facility (MFFF) are currently being designed for construction at the Savannah River Site (SRS) (Siskin, 2002).
The inventory includes approximately 17 metric tons (MT) of plutonium in the forms of scraps and residues, including very impure materials with high halide content. Until canceling the project in early 2002, DOE intended to immobilize this impure Pu-239 in a ceramic material for co-disposal with vitrified high-level waste (DOE, 2000c). The immobilization facility was to have been built at SRS and used in conjunction with the planned pit disassembly facility, MFFF, and the currently operating Defense Waste Processing Facility, which is vitrifying high-level tank waste at the site. The immobilization facility was cancelled primarily for economic reasons (Siskin, 2002).
Coincident with canceling the immobilization facility, DOE modified the MFFF to include an “alternate feedstock” capability, which is intended to allow processing of most of the 17 MT. However, the technical basis for this plan remains uncertain. According to an assessment by the Defense Nuclear Facilities Safety Board (DNFSB), “While there is a reasonably good assurance of sound disposition paths for 36 MT of these [Pu-239] materials, the disposition paths for approximately 17 MT of metal, oxides, and fuel have considerable uncertainty. Of this latter group, about 6 MT of material has no disposition path” (DNFSB, 2002, p. 3-8).
Disposition Options and Challenges
DOE’s disposition options for surplus Pu-239 include the following:
-
storage according to the DOE 3013 Standard for up to 50 years;
-
fabrication into MOX fuel;
-
disposal as transuranic (TRU) waste in the Waste Isolation Pilot Plant (WIPP); and
-
disposal along with high-level waste and spent fuels, for example, in the planned Yucca Mountain repository.
Extended Storage
At the end of the Cold War, the redirection of mission and scheduled closure of plutonium processing facilities in the DOE weapons complex left significant quantities of Pu-bearing materials in interim or ill-defined status. Normal processing operations would have moved these materials to final disposition or utilization. The DNFSB Recommendation 94-1 (DNFSB, 1994; DOE, 1994a) led DOE to
establish a program to stabilize, secure, and process these materials to enable closure of plutonium facilities that no longer have a sustainable mission. This program continues to sponsor research and to develop and implement techniques to convert and stabilize plutonium-bearing materials for storage of up to 50 years. The program’s goal is to have materials with greater than 30 weight percent plutonium in a stable, solid form that meets the DOE 3013 Standard.
The main challenge in implementing the 3013 Standard is reduction of moisture to within acceptable limits in the plutonium-bearing materials. The problem with moisture contained within radioactive solids is that in a confined, sealed package pressure can potentially build as a consequence of radiolytic decomposition of water and perhaps other molecules. In addition, gases produced in these sealed containers are expected to contain enough hydrogen gas to be of concern from safety and transportation standpoints. Plutonium-bearing materials that are less pure than metals and oxide frequently contain highly variable and poorly characterized constituents. These materials increase the challenge for processing to meet the 3013 Standard.
MOX Fuel Fabrication
For eventual disposition of the inventory, as much of the excess material as possible—including all materials currently in pit form—will be processed and fabricated into MOX fuel for once-through use in commercial nuclear reactors. The DOE has solicited specifications from European nuclear fuel manufacturers who routinely perform MOX recycling. Following use in commercial nuclear reactors, the spent fuel is expected to be packaged and shipped to Yucca Mountain (assuming it is licensed and constructed) for disposal.
The technical basis for MOX fuel has been fairly well established through work by DOE and its predecessor agencies (Freshley, 1973; Pedersen, 2002). The barriers to a substantial program are economic and institutional. First, the fuel fabrication facilities used for current light water reactors (LWRs) cannot accept MOX feed material because of radiation safety and safeguards requirements. The MOX facility ends up much more expensive in both capital and operating costs for equivalent throughput. Second, the fuel rods and assemblies will have much more stringent handling and shipping requirements than clean LWR fuel. Only when they reach the fuel canal of the reactor site will they be handled using techniques familiar to today’s operating personnel, who are used to handling both clean and partially burned LWR assemblies in refueling operations. Third, the licensing basis for the fuel must account for the differences in reactor transient and control characteristics, accident behaviors and consequences, and safeguards require-
ments. The facility development, operational characteristics, and other important aspects related to developing a significant MOX program are currently being addressed by a number of research activities (Anderson, et al., 2002; Johnson and Brabazon, 2002; Naugle, 2002).
DOE has estimated that only about 2 of its 17 MT of impure plutonium-bearing materials cannot be sufficiently purified (for technical or economic reasons) to meet MOX feed specifications (Siskin, 2002). A representative distribution of some of these less pure plutonium materials, stored at Hanford (most of which were transferred from Rocky Flats, Colorado), is shown in Figure 3.3 (Venetz, 2002). The variety of materials, expressed as a function of plutonium content versus number of items, is from an inventory of about 900 items containing approximately 0.8 metric tons of plutonium with a total weight of 1.1 metric tons. Of note are the 79 items with plutonium contents less than 50 weight percent. In addition, there are also significant numbers of inventory items with plutonium contents ranging from 50 to 70 weight percent. Most of the analyzed samples have high chloride concentrations. Furthermore, many (if not all) of these materials have silicon concentrations ranging from 4 to 10 weight percent. The chemical properties of chloride and silicon, respectively, are detrimental to stor-
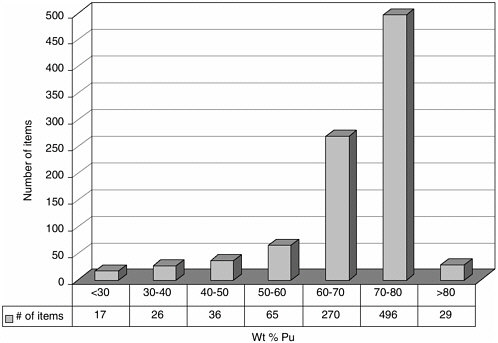
Figure 3.3 An inventory of impure plutonium oxides stored at Hanford shows that some materials may be too poor in plutonium for economical conversion to mixed oxide fuel but too rich for disposal as transuranic waste. Research may help improve both the technologies and the basis for decision making for dealing with such materials. Source: Venetz, 2002.
ing plutonium in the 3013 cans and processing it to make MOX fuel (WSRC, 2002).
Disposal in WIPP
Besides conversion to MOX fuel, DOE’s other planned disposition route for excess plutonium is disposal as TRU waste at the WIPP in southeastern New Mexico. DOE currently has some 111,000 m3 of TRU waste in retrievable storage awaiting shipment to the WIPP. Most of this volume consists of contaminated clothing, equipment, and process wastes that contain relatively small amounts of plutonium. Plutonium-bearing scraps and residues that meet the repository’s waste acceptance criteria (WAC) can also be disposed. The WAC include nuclear safety limits of less than 200 “fissile gram equivalents” of Pu-239 per 55-gal drum of waste; no compressed gases, liquids, reactive or corrosive wastes; and no pyrophoric materials (DOE, 2002f).
As an example of increased WIPP utilization, DOE has recently amended a record of decision to allow an additional 0.97 MT of low assay oxides containing about 0.18 MT of plutonium to be sent to WIPP (DOE, 2002c, 2002d). These materials are similar to other plutonium residues that are already being sent to WIPP from Rocky Flats. Because of their impurities, DOE considers that these materials would be impractical to ship to SRS for fabrication into MOX fuel (see previous section). However, proposals to dispose of unanticipated large amounts of plutonium are likely to encounter political resistance (Domenici, 2002).
Co-disposal with Defense High-Level Waste
The DNFSB noted that there are uncertainties in DOE’s current plans for its 17 MT of impure plutonium. The committee believes that as a practical matter some scraps and residues will fall in an ill-defined category of containing too many varied impurities for conversion to MOX and being too concentrated to meet the WIPP criteria. For these materials, DOE could revert to its earlier option of co-disposing some of this plutonium with defense high-level waste and spent fuels (NAS, 1994).6 In the earlier option, incorporating the plutonium into a ceramic was proposed to meet waste acceptance criteria and provide resistance to theft (NAS, 2000).
The principal challenge in formulating crystalline ceramic hosts is radiation-induced transformation from a crystalline to an amorphous state (amorphization), mainly the result of self-irradiation damage asso-
6 |
A review of research and development in support of this option is at http://www.llnl.gov/str/gray.html. |
ciated with the alpha-particle emission (a-decay) of incorporated actinides, which can lead to large volume changes, increased surface area due to cracking, and enhanced corrosion rates. An acceptable host will have to accommodate at least a 10 percent loading of Pu to limit the overall waste volume, and remain stable for several hundred to several thousand years.
Radiolytic degradation of certain host ceramics, principally silicates and some phosphates, is an issue because cumulative ionization doses can reach 109 Gy in about 300 years for a 10 percent host loading of Pu-239, and > 1011 Gy after 100,000 years. Over the past decade, DOE research efforts for plutonium disposition in crystalline hosts have focused on titanates, such as brannerite (UTi2O6), which are not as susceptible to radiolysis. Such structures eventually amorphize by accumulation of damage zones produced during a-decay by energetic recoil of the actinide nucleus (kinetic energy ~100 keV, range ~40 nm). Irradiation-induced amorphization competes with simultaneous recrystallization and typically occurs at a cumulative displacement dose of ~0.1 to 1 displacement per atom below a critical temperature that is often well above room temperature.
Research Needs and Opportunities
The EMSP should support research to help maximize the portion of DOE’s excess plutonium inventory that can be used as MOX fuel and that will support the scientific basis for disposal of impure plutonium not suitable for MOX. Research should include fundamental chemistries for storing and purifying plutonium, modeling of MOX fuel performance to help ensure reactor safety, and devising high- integrity, theft-resistant forms for disposal.
There are opportunities for the EMSP to partner with other DOE offices (National Nuclear Security Administration, DOE Office of Environmental Management) to support plutonium storage and fabrication into MOX fuel. Ongoing projects in other offices could be extended to improve scientific understanding of long-term corrosion, gas generation, and moisture analysis relevant for storage of plutonium-bearing materials, process analytical chemistry and materials characterization for MOX fuel fabrication, and nondestructive assay technique improvements for use in high-radiation environments. Developing plutonium immobilization matrices that would resist illicit recovery of the plutonium or its dispersion is desirable.
For less pure materials, characterization and separation of undesirable impurities are needed to render more of the material suitable for MOX fuel. Research in support of MOX fuel fabrication programs may allow greater flexibility in incorporating a broader range of materials than current MOX fuel specifications allow. For material not destined for MOX fuel or WIPP7 there are opportunities for research toward developing highly durable forms for co-disposal with high-level waste and spent fuel. Finally, there is potential for crosscutting research toward stabilization of both spent fuel and plutonium residues for storage, as well as for eventual disposal.
Stabilization for Storage and Transportation
The interim processing end point for materials with greater than 30 weight percent plutonium is a solid material that has been processed according to the DOE 3013-2000 Standard (DOE, 2000b). The transportation of stabilized plutonium-bearing solids follows from the requirements of the Code of Federal Regulations, Article 49, concerning transportation of containers bearing plutonium materials, that they not contain pyrophoric or potentially explosive contents (Bailey et al., 2000). These requirements are satisfied by processing and packaging the plutonium according to the 3013 Standard. The stabilization requirements and the container design represent a balance between achieving consistently low moisture content (to avoid container pressurization via radiolysis) and containing a “worst case” internal pressure. Surveillance programs have not, however, verified the high pressures predicted by the pressure equation currently used in the 3013 Standard (Berg et al., 2002; Duffey and Livingston, 2002).
There are opportunities for research on long-term corrosion and gas generation mechanisms inherent in these sealed, plutonium-bearing materials to ensure that they remain safe regardless of the duration of storage while reducing unnecessary conservatism in the standard. This research would involve fundamental study of atomic interactions on the solid surfaces of the stored material. Material forms of concern include those with relatively high salt residues, for example, plutonium mixed with residual magnesium and calcium compounds and other solids thought to be largely oxides and hydroxides, resulting from calcination
in air environments at 950 °C. The hygroscopic property of these materials dictates that some moisture will be present following 3013 Standard canning in most plant operations.
Research into novel, noninvasive (in situ) pressure and hydrogen content-measuring technologies is of potential utility as a cost-efficient means to track and identify future problems in sealed materials in interim or long-term storage. In addition, there are crosscutting issues concerning interaction of water with uranium and spent nuclear fuel elements as well as with plutonium. For example, are the mechanistic details of gas generation common to many of these actinide surfaces? Are there molecular or material science issues that are unique to each element or material type?
Regardless of whether processed material is intended for conversion to MOX fuel or simply stored and secured for time intervals as long as 50 years, understanding the corrosion aspects of the storage system has high pragmatic value (Kolman, 2001). Corrosion may be increased due to elevated temperatures that are likely to occur during storage for two reasons: the multi-barrier 3013 storage canister tends to insulate the plutonium, and the poorer grades of plutonium are contaminated with isotopes (Pu-240, Pu-241, Am-241) that have shorter half-lives and thus produce more heat than pure Pu-239. Whether these materials can be stored at potentially elevated temperature remains an unresolved question and represents a gap in technical knowledge. Open questions regarding the combined effects of conventional radiation-assisted corrosion in these long-term storage environments need to be addressed through fundamental research programs.
There are other less fundamental, but nevertheless important, research opportunities to improve the plutonium calcination process. Furnaces and furnace components frequently exhibit spectacular and debilitating corrosion effects that seriously affect processing timelines, and secondarily, site closure schedules. These issues have been identified in disposition planning documents driven by specific site concerns (WSRC, 2002) but are common to many sites attempting safe processing and securing nuclear materials.
In addition, material protection, control, and accounting (MPC&A) are always of concern for plutonium-bearing materials to ensure that diversion or theft of weapons-grade materials does not occur. Although conventional nondestructive analysis techniques are frequently used to verify the fissile content of materials, there are opportunities for research into more efficient and rapid MPC&A technologies. As noted in Chapter 7, the nation’s supply of Pu-244, used as a tracer in isotope dilution mass spectrometry for control and accountability determinations, is severely limited.
Conversion to MOX Fuel
DOE’s decision to convert its 17 MT of impure plutonium to MOX presents a variety of research needs and opportunities. These “alternative feedstocks” will require processing and polishing operations to remove specific elemental and fission-product neutron poisons detrimental to their use in reactors and to remove constituents that are incompatible with long-term stability of fuel-rod cladding. Specific examples relating to conversion of weapons-grade plutonium include removal of gallium down to very low concentrations and removal of silicon to acceptable levels (SROO, 2002).
Techniques and methods for processing impure plutonium (scrap recovery) have been used successfully since the early 1950s (see Appendix A). The challenge is to extend these methods to economically recover increasingly impure portions of the 17 MT inventory. Along with research to help ensure cleanup of feedstock to established impurity limits, research that might lead to relaxing the limits for some impurities could have a substantial economic benefit.
Additional basic process engineering research is also warranted to convert plutonium materials into suitable MOX fuel in the most efficient manner minimizing cost, time, and facility usage. A specific question is whether multiple batch operations are a more efficient means of processing both routine and nonroutine feedstocks than continuous flow-through processing. Rapid low-cost analytical means of determining impurity levels of feed materials will be required to answer such a question. In addition, accurate determination of impurity levels in the highly radioactive environment of the plutonium matrix poses unique challenges for the analytical chemist. Current means of determining impurity levels of interest at sub-ppm levels require arduous dissolution procedures followed by atomic emission spectroscopy techniques. Improvements in this arena are needed to make rapid decisions about process efficiency.
Another key research area that would benefit MOX fuel processing includes nondestructive analysis (assay) methods (NDA). Improved methods can facilitate examination of nuclear fuel to assure that materials are not being diverted for clandestine purposes. Advantages of NDA techniques include remote operation and analysis, lower safety risk, and analytical determinations in difficult-to-assess or field environments. Nuclear safeguards and surety programs have historically provided the bulk of the funding to develop and implement these techniques, but there is opportunity for the EMSP to enhance the fundamental scientific basis for new techniques.
Alternatives for Co-Disposal with High-Activity Waste
The committee believes that there is sufficient uncertainty in current plans to convert all excess plutonium to MOX fuel or to dispose of it in the WIPP to warrant further research to support disposal in a geological repository designed for high-activity waste. Crosscutting research could also provide scientific support for disposing of spent reactor fuels, discussed in Chapter 4, and other actinide elements, discussed in Chapter 7.
Ceramic Matrices
Research into immobilizing plutonium from difficult-to-process materials classes is warranted from the standpoint of long-term stable disposition and may include development of novel material types and technologies. A comprehensive set of research recommendations for evaluating alternative crystalline wasteforms for plutonium disposition with respect to radiation stability emerged from an expert panel convened under the auspices of the DOE Council on Materials Science (Weber et al., 1998). The most promising actinide host phases cited include the structure types of pyrochlore, zirconolite, zircon, apatite, perovskite, titanite, monazite, zirconia, and sodium zirconium phosphate (NZP).
Stabilization of plutonium in crystalline solid forms is advantageous because the radionuclide is predictably coordinated in well-defined atomic sites in a durable solid that has been demonstrated—in many cases in geological analogues persisting over millions or billions of years—to resist release of the radionuclide in hydrologic corrosion conditions. Containment at well-delineated atomic sites in a crystal structure can supplement the geological barrier in providing assured isolation of very long-lived radioactive materials.
Crystalline ceramics may also be designed and synthesized to accommodate a specific radionuclide with optimal retention. Materials can be designed to obtain a desired radiation response, whether by building in certain defect structures to accommodate displaced atoms or providing ion sizes and charges and host structures that favor retention of crystallinity (and its predictable radionuclide coordination) when atoms undergo radiation disordering. It is the complexity and latitude of ceramics that makes these approaches possible and that provides opportunities for basic research. For example, it may be that the ideal radiation-stable plutonium host is an “amorphous” phase resembling the radiation-disordered phase that will eventually result and thus exhibit few further physical, chemical, or structural changes upon subsequent radiation disordering. Such an engineered phase might have predictable actinide coordination and short- and medium-range order
little different from those of the crystal (Hobbs et al., 1999). Adding U-238 to the ceramic matrix may provide long-term criticality control by isotopic dilution as Pu-239 eventually decays to U-235.
Structural derivatives of the fluorite structure are potential crystalline hosts for plutonium because of the ability to readily incorporate actinides possessing fluoritic structures (UO2, ThO2, PuO2). Furthermore, these structural types can readily incorporate ZrO2 (of interest because of its use as an inert matrix for plutonium burnup in a reactor or accelerator-based neutron source). Additional structural types either under study or of potential interest include perovskites (e.g., CaTiO3); zircon (ZrSiO4); the silicotitanate sphene (or titanite, CaTi(SiO4)O); the phosphate compounds monazite (LnPO4); apatite (Ca4-x RE6+x(SiO4)6-y(PO4)yO2); NZP (NaZr2(PO4)3); and the A2B2O7 pyrochlores in which the A-site contains large cations (Na, Ca, rare earths, actinides); and the B-site smaller high-valence cations (Nb, Ta, Ti, Zr, Hf, Sn, Fe3+, Pb). Zirconolite (CaZrTi2O7), a fluorite derivative closely related to pyrochlores, is the primary actinide host phase in the polyphase Synroc crystalline high-level waste form. Pyrochlores and related derivatives are especially attractive as long-term storage hosts because of the large range of elements (and actinides) they can accommodate, which is a particularly useful property in immobilizing plutonium from impure plutonium sources.
Recent studies (Sickafus et al., 2000; Wang et al., 1999) have shown that certain plutonium-accommodating titanate-based lanthanide pyrochlores can be synthesized and exhibit remarkable radiation resistance against amorphization by substituting Zr for Ti. Because pyrochlores are fluorite structure derivatives, disordering the cation sublattices yields an average defect fluorite structure; the fluorite structure class is the most resistant to amorphization because of the huge redundancy in structural constraints (Hobbs et al., 1996, 1999). The closer the initial pyrochlore structure is to the fluorite structure, the more stable the cation-disordered structure is toward subsequent amorphization; this observation is more pronounced for the pyrochlore lanthanide zirconates than for the lanthanide titanates, and particularly so for actinide substitutions.
Several other countries have ongoing research in crystalline ceramics. These provide opportunities for international collaborations. The Australian National Science and Technology Organization has a research program that is now several decades old, which continues to investigate titanate-based waste forms. The most recent efforts have been to develop a pyrochlore (Gd2Ti2O7) for the immobilization of plutonium. In Russia, a number of institutes work on ceramic waste forms (many supported by DOE). The principal structure types investigated include zircon, zirconolite, pyrochlore, NZP, and garnet. British
Nuclear Fuels, Ltd., is funding a number of university centers to develop a variety of waste forms. Researchers at Cambridge are studying radiation effects in zircon (a possible host phase for plutonium), perovskites, and Zr-pyrochlores. China and Japan have generally modest programs on waste forms, mostly investigating titanate phases.
Under French law, research programs have until 2006 to provide the necessary data to support the development of a waste disposal strategy. At present, the crystalline waste forms under consideration have been narrowed to zirconolite, monazite, apatite, and a thorium diphosphate-phosphite. In addition, the French still maintain a very active fundamental research program on the properties of glass waste forms.
Alternative Processing
A recently developed process (INEEL, 2001) involves the treatment of plutonium-containing salts with an electro-dissolution step that transports and deposits uranium (and plutonium if desired) in a lithium chloride-potassium chloride eutectic salt. Periodically the salt is disposed as high-level waste due to accumulation of fission products and other impurities, including plutonium if it is not recovered. The salt is ground and hot-blended with a zeolite, and the mixture blended with glass particles and roasted. Process control variables include types and particle sizes of salt, zeolite, and glass, and temperatures of contacts and roasting conditions. It is important to know, however, if the identity of the LiCl-KCl residual components is retained in the final waste form. While the product appears to meet waste acceptance product specification criteria in leach and durability tests as produced, at short times or with low activity surrogates, it may not do so in the long term with the radiation field of Pu-239, particularly if the chlorides are retained as alkali halide compounds and do not dissolve into the zeolite or glass matrices. Alkali halides are perhaps the most radiation sensitive of all materials and undergo efficient radiolytic decomposition in an ionizing radiation field; substantial decomposition would be expected in repository conditions in several hundred years for a 10 weight percent plutonium loading. Even if the salt is dissolved into the glass or the zeolite, both will also undergo substantial structural changes from ionizing and displacive radiation fields of the plutonium a-decay, and their chemical durability will change. Long-term stability for any new waste form in a radiation field needs to be investigated.
Canister Integrity
Once a plutonium-bearing material has been classified as a waste and is processed into a stable form, the integrity of its containment system needs to assured. Radiation-induced corrosion and other potential release mechanisms need to be determined. Although preliminary needs and critical issues have been identified in a number of recent
communications (Wang et al., 1999; Weber et al., 1998) additional experimental work will undoubtedly be required for alternative material forms. Dimensional changes in the solid waste form, due to potential amorphization of crystalline components or restructuring of any glass components, are one such issue for the integrity of the waste form itself, as well as integrity of the encapsulating container. Anisotropic dimensional changes are known to cause disaggregation of polycrystalline waste forms through grain boundary and transgranular fracture. This is less a problem for fine-grained material (submicrometer to micrometer grain sizes) but becomes a significant effect for larger grain sizes; Synroc, for example, is typically produced with 10–100 µm grain sizes. Measurements of internal stress developing with irradiation in accelerated tests with short-lived isotopes, for example using X-ray diffraction-based or Raman-based stress measurements used for assessing stresses developing in oxidation scales, need to be carried out. Processing methods for producing fine-grained polycrystalline assemblages of host phases incorporating appreciable plutonium or other actinides need also to be researched.