3
Unintended Effects from Breeding
This chapter explores the likelihood of unintended effects from diverse methods of genetic modification of plants and animals (see Operational Definitions in Chapter 1). Specifically, it discusses unexpected outcomes of breeding methods used to develop a food crop or strain and unexpected or unintended effects recorded in the scientific literature. It also includes analyses of methods intentionally used for modifying food sources and comparing the likelihood of unintended changes resulting from the use of genetic engineering versus other methods of genetic modification discussed in Chapter 2.
BACKGROUND
Novel gene combinations arising from the genetic manipulation of existing genes through conventional breeding techniques may introduce unintended and unexpected effects. However, through the breeder’s selection process, the genetic lines that express undesirable characteristics are eliminated from further consideration, and only the best lines—those that express desirable characteristics with no additional undesirable agronomic characteristics, such as increased disease susceptibility or poor grain quality—are maintained for possible commercial release. Although plants and animals produced from conventional breeding methods are routinely evaluated for changes in productivity, reproductive efficiency, reactions to disease, and quality characteristics, they are not routinely evaluated for unintended effects at the molecular level. New varieties of food crops, other than those produced using recombinant deoxyribonucleic acid (rDNA) technologies, are rarely subjected to toxicological or other safety assessments (WHO, 2000). Previous National Academies committees have addressed the question of
whether unintended effects arising from the use of rDNA-based technologies in food production and the risks potentially associated with them differ in nature and frequency from those associated with non-rDNA-based breeding methods (NRC, 1987, 2000, 2002).
There is a considerable amount of data compiled and available in the scientific literature addressing issues related to genetically modified (GM) and genetically engineered (GE) plants, including health and environmental impacts. In contrast, development of transgenic animals is a relatively new area of biotechnology, and so the amount of data collected and reported for GM animals is less than that for plants. However, the application of genetic modification techniques and the potential for unintended adverse effects are similar for both plants and animals, and much of the information obtained from plants can be applied to questions of concern in the genetic modification of animals.
PLANT BREEDING
Conventional Plant Breeding
Conventional plant production occasionally generates foods with undesirable traits, some of which are potentially hazardous to human health. Most crops naturally produce allergens, toxins, or other antinutritional substances (see Chapter 5). Standard practice among plant breeders and agronomists includes monitoring the levels of potentially hazardous antinutritional substances relevant to the crop. For example, canola breeders monitor levels of glucosinolates in breeding lines under consideration for prospective commercial release, while potato breeders monitor for glycoalkaloid content. If a particular breeding line generates too much of an undesirable substance, that line is eliminated from consideration for commercial release.
In the United States, the plant breeding community is largely self-monitored. Regulatory agencies do not evaluate conventional new crop varieties for health and environmental safety prior to commercial release. Some other countries require government agencies to conduct premarket evaluations for new crop varieties, both conventional and biotechnology-derived. Canada has a “merit system” for the commercial release of new varieties of major field crops, in which candidate varieties are grown in government-administered field trials. Performance data from these trials, related to agronomic factors, disease resistance, and food quality characteristics, are compiled for all candidate and standard commercial varieties. The data are collected from multiple locations over multiple years, as cereal chemists analyze the grain for chemical and nutritional composition and plant pathologists conduct tests to determine reactions to relevant diseases. These data are then evaluated by a team of experts from industry, government, and universities.
The breeder of each candidate variety must convince these experts that it is competitive and worthy relative to other commercially available varieties of that
crop, based on the performance data from the trials. Only if the committee agrees is the candidate variety allowed to be registered as a new commercial variety. If the variety does not perform within prescribed parameters for all characteristics, it is not commercially released (CFIA, 2003).
Unintended Effects of Conventional Plant Breeding
Naturally Occurring Toxins
All foods, whether or not they are genetically engineered, carry potentially hazardous substances or pathogenic microbes and must be properly and prudently assessed to ensure a reasonable degree of safety. Furthermore, all crop strains, including organic strains, potentially express traits generated by various forms of induced mutagenesis. (Under organic regulations, radiation breeding and induced mutagenesis are acceptable, but irradiation of the final food itself is not. For more information on organic regulations, see USDA-AMS, 2001.)
History provides examples of traditional breeding that resulted in potentially hazardous foods (see Box 3-1). Solanaceous (tobacco family) crops, such as potato and tomato, naturally produce various steroidal glycoalkaloids. These substances are toxic not only to humans, but also to insects and pathogenic fungi. During the course of ordinary plant breeding assessments, breeding lines with increased levels of glycoalkaloids may be identified by the breeder as showing superior insect or disease resistance and retained for possible commercial release. The elevation of glycoalkaloid levels responsible for the pest tolerance may not be noted until people become ill from consuming the foods.
Tomatine, a glycoalkaloid naturally present in tomatoes, can be produced in hazardous quantities in certain conventionally bred varieties. Ordinarily, alpha tomatine is present in immature tomato fruit, but is degraded as the fruit matures, so that by the time the fruit ripens to the preferred stage for human consumption, tomatine content is reduced to safe levels. Nevertheless, levels of naturally occurring tomatine in ordinary tomatoes bred using conventional methods can vary considerably, primarily based on maturity, type, and environmental growing conditions (Gilbert and Mohankumaran, 1969; Leonardi et al., 2000). In this respect, environment is more responsible for food hazards than genetic makeup or breeding method.
Another example of a possible effect is the unintended elevation of glycoalkaloid content in potatoes. All potatoes produce the toxic glycoalkaloid solanine, but mature potatoes from most cultivars have amounts so small as to be nonhazardous. However, some varieties produce more than others, and certain environmental stimuli, such as growing or storage conditions, can cause potentially hazardous increases in solanine content, even within a usually safe cultivar (Concon, 1988). For example, potatoes exposed to sunlight turn green, making them particularly prone to high solanine content. Dark-skinned varieties are less
BOX 3-1 Puffer fish, chile peppers, and mustard all are traditional foods that humans have learned to consume in moderation to minimize adverse health effects. But what about foods entirely new to the human digestive tract? Although some have designated (GE) foods as completely novel, the GE varieties currently approved and on the market are of the same composition as other foods. Corn oil, for example, is chemically identical regardless of the breeding method used to develop the corn variety. In recent history, the closest example of a new food might be kiwi fruit. Originally it was an edible but unpalatable plant producing small, hard berries in China. Breeders in New Zealand developed what we know now as kiwi fruit (Actinidia deliciosa) into a food during the twentieth century, and commercialized in the United States during the 1960s. There does not, however, appear to be any official record of a premarket safety analysis of the fruit. As a consequence, some humans who were not previously exposed to kiwi fruit developed allergic reactions. Recently, well after commercial release, the responsible allergenic protein (actinidin) was isolated and characterized (Pastorello et al., 1998). |
likely to turn green than light-skinned varieties, but in either case environment seems to be more important to solanine production than genetics.
Certain potato lines have been found to express greater disease- or pest-resistance, and they have been selected as superior, not always with favorable or intended results. The most notorious such selection was the Lenape potato, which was developed using conventional breeding methods (Akeley et al., 1968). After a successful commercial launch, it was found to have dangerously elevated solanine content in the tubers and was removed from the market (Zitnak and Johnston, 1970). More recently, a similar high-solanine potato variety was detected and withdrawn from the market in Sweden (Hellanäs et al., 1995). In this case, the potato was a heritage variety, developed in the United Kingdom during the nineteenth century, but superseded by another variety due to its susceptibility to disease (Kuiper, 2003). Nevertheless, it became popular in Sweden under the name “Magnum Bonum,” until its predilection for overexpressing solanine resulted in its commercial demise (Hellanäs et al., 1995).
In spite of occasional problems with the consumption of potato glycoalkaloids, conventional breeders continue to increase the glycoalkaloid content in the leaves to take advantage of its pest- and pathogen-deterrent properties. Consequently, the U.S. Department of Agriculture (USDA) recommends, but does not require, a limit for glycoalkaloid content in new potato varieties (Sinden and Webb, 1972).
Interestingly, the Lenape (also known as breeding line no. B5141-6) potato has been found to express some useful attributes, such as high solids content. Thus Lenape continues to be used successfully as a parent in conventional breeding programs, providing its genes to new commercial potato varieties, such as Atlantic and Denali. Progeny with genes providing the high solids content are selected or maintained, and the genes responsible for high solanine content in the tubers are selected against or rejected. Additionally, Lenape has been transformed with a genetic construct containing the solanidine glucose-adenosine diphosphate glucosyltransferase (SGT) transgene in the antisense direction, which is designed to interfere with the solanine biosynthesis (Moehs et al., 1997). In field trials, several transgenic Lenape-derived lines expressed substantially less solanine than the parent Lenape, apparently due to antisense expression, an rDNA method for “turning off” an undesirable gene (McCue et al., 2003).
Several breeding programs are developing potatoes derived from conventional crosses between the ordinary potato Solanum tuberosum and relatives of other species, such as S. acaule (Kozukue et al., 1999) or S. chacoense (Sanford et al., 1998; Zimnoch-Guzowska et al., 2000). These are conventional breeding programs in which genes from two different species are exchanged. The intent of these conventional breeding programs is to generate potato varieties with new beneficial features, while minimizing deleterious traits from the foreign species.
Breeders typically monitor levels of toxins in plants that are known to naturally contain them, even though such monitoring is only voluntary in the United States. However, an unexpected and unintended problem may result when combining different species because thousands of genes would be interacting, not just one or two genetic elements of interest. For example, hybrids of S. tuberosum and S. brevidens produced not only the usual glycoalkaloids, but also the toxin demissidine, which is not produced in either parent (Laurila et al., 1996). This singular result shows that non-genetic engineering breeding methods can have unintended effects and generate potentially hazardous new products.
Any time genes are mutated or combined, as occurs in almost all breeding methods, the possibility of producing a new, potentially hazardous substance exists. Conceivably, similar outcomes could result from using rDNA to transfer specific genes from S. brevidans to S. tuberosum, giving rise to hybrids expressing the novel toxin demissidine. In either case, the hazard lies with the presence of the toxin, and not with the method of breeding. Genetic engineering could also be used to transfer only the beneficial genes from S. brevidans, leaving behind the genes responsible for the novel toxin.
Another example of a toxic compound from traditional crops is psoralens in celery (see Box 3-2). Celery naturally produces these irritant chemicals that deter insects from feeding on the plant and also confer protection from some diseases (Beier and Oertli, 1983). Celery plants with an elevated expression of psoralens will suffer less damage from disease and insect predation and have more aesthetic appeal to consumers, who tend to reject insect- or disease-damaged produce.
BOX 3-2 Genetic engineering has raised the question of whether its products create unintended health effects for consumers, but how do conventionally bred products compare along the continuum of genetic modification? Celery provides an interesting case, as it contains naturally occurring toxic chemicals called psoralens, which are secondary metabolites found in a variety of fruits and vegetables, and members of the class of compounds called furanocoumarins (Diawara and Kulkosky, 2003). The psoralen in celery provides it with a biological defense mechanism of sorts—if it suffers disease or has been bruised, the celery plant can produce up to 100 times the level of psoralen that it typically contains, thus protecting itself from attacks by pests. The production of psoralens also depends on factors such as temperature, season and, particularly, availability of sunlight (Diawara et al., 1995). Psoralens temporarily sensitize human skin to longwave ultraviolet radiation. Consequently, they have been used for more than 25 years as a component in phototherapy—a means of treating acute skin diseases (New Zealand Dermatological Society, 2002). However, psoralen in celery has been implicated in cases of skin irri-tation, such as dermatitis, among farm workers and other handlers of these plants, and studies have suggested a potential correlation between psoralens and cancer in laboratory mice (Beier, 1990). Moreover, celery cultivars produced using conventional breeding methods—intended to enhance insect-resistance and aesthetic appeal to consumers through increased production of psoralen—have been associated with cases of dermatitis among grocery workers, as well as further cases of photosensitivity among farm workers handling these plants (Ames and Gold, 1999). This form of dermatitis, or “photodermatitis,” has been observed as far back as 1961 in field workers who had handled celery infected with the disease pink rot (Birmingham et al., 1961). The Centers for Disease Control and Prevention reports cases dating back to 1984 of severe skin rashes among laborers who handled celery on a regular basis. The initial onset of these cases spawned a study by the National Institute for Occupational Safety and Health, in which there appeared to be a relationship between the handling of celery and prolonged exposure to sunlight. In the study that was undertaken in 1984, a number of grocery workers who handled celery on a regular basis also had used a tanning salon, suggesting to researchers that the ultraviolet light from tanning had exacerbated the reactions of the workers’ skin in response to the celery they had handled (CDC, 1985). Exposure to elevated levels of psoralens remains a problem for laborers such as field workers, as constant exposure to sunlight—combined with the handling of vegetables such as celery—can induce reactions such as those occurring in the skin. Psoralens continue to be regarded as naturally occurring toxicants (Beier, 1990). |
As a result, breeders may observe healthy, undamaged celery lines and select them for commercial release. Unfortunately, workers who harvest high psoralen-producing celery or pack it in grocery stores have, on occasion, developed severe photodermatitis, especially when they are exposed to bright sunlight and the celery is infected with pathogens (Berkley et al., 1986; Birmingham et al., 1961; Finkelstein et al., 1994). There are differences in psoralen content from one variety to another (Beier, 1990; Diawara et al., 1993), but environment seems to have the greatest influence on psoralen production (e.g., Diawara et al., 1995).
Mutations
Mutations, defined as any change in the base sequence of DNA, can either occur spontaneously or be induced, and both methods have produced new crop varieties. Most mutations are deleterious and therefore useless for breeding purposes. However, a mutation can result in desirable traits and may be selected for breeding. Spontaneous mutations, also called “sports” by horticulturists, are by definition not induced, so breeders wait a long time to see mutants arising from this process, and even longer to see useful ones. Most mutation breeders induce random changes in DNA by using ionizing radiation or mutagenic chemicals, such as ethyl methane sulfonate, to increase the rate and frequency of the mutation process.
In spite of these intrusive methods, induced mutagenesis is considered a conventional breeding technique. Food derived from mutation breeding varieties is widely used and accepted. Organic farming systems permit food from mutated varieties to be sold as organic. In the United States many varieties have been developed using induced mutagenesis, such as lettuce, beans, grapefruit, rice, oats, and wheat. The Food and Agriculture Organization of the United Nations/ International Atomic Energy Agency Mutant Cultivar Database (FAO/IAEA, 2001) lists more than 2,200 varieties of various species worldwide that have been developed using induced mutagenesis agents, including ionizing irradiation and ethyl methane sulfonate. However, the database does not include spontaneous mutations, cell-selected mutants (Rowland et al., 1989), or somaclonal variants (Rowland et al., 2002).
There is no mandated requirement to list new mutant varieties with the database. However, there do not appear to be outstanding examples of mutant varieties with documented unexpected effects beyond what the mutant was selected for, despite the expectation that mutant varieties may possess and generate more unexpected outcomes than ordinary crosses because of the unpredictable and uncontrollable nature of nontargeted mutations. Furthermore, there do not appear to be any examples in which mutant varieties were removed from the market due to unintended or unexpected adverse incidents.
Genetic Engineering in Plants
As for conventional breeding techniques, the genetic engineering (GE) breeding and selection process is largely self-monitored and only new varieties of rDNA crops are subjected to assessment prior to commercialization. Evaluation of GE food products for other countries is described above, in the section Conventional Plant Breeding.
Genetic engineering methods are considered by some to be more precise than conventional breeding methods because only known and precisely characterized genes are transferred. In contrast, conventional breeding involves transferring thousands of unknown genes with unknown function along with the desired genes. Similarly, in mutation breeding hundreds or thousands of random mutations are induced in each mutated line.
Plant breeders take a variety of methods used to introduce desired traits into consideration during selection. The location where DNA expressing the desired traits is inserted into the host genome in rDNA technology may be immaterial—for example, if an insertion is made in an inappropriate place, the transformed plant is eliminated or selected against by the breeder, who will then select another plant with a preferable locus of insertion for continued evaluation and development as a new variety.
The number of different lines developed by breeders varies according to the crop, the desired trait, and the choice of the breeder, but is it not unusual to start with evaluations of 2,000 “sister” lines from a cross of two parents to develop just one new variety. Thus 99 percent of sister lines are eliminated over the several years of evaluation, for various reasons. These include poor expression of the desired trait, poor yield performance, increased disease susceptibility, or even a lack of visual and tactile appeal, which is a largely subjective and arbitrary designation, but an important criterion nevertheless.
Genetic engineering techniques require fewer lines or transformation events because the desired trait is known and identified early. Consequently, the evaluations—which still take several years—focus on eliminating any unstable lines or those with deleterious characteristics.
In contrast to breeders using other techniques, genetic engineering breeders typically prefer to start with a small number of plants and then select only a few. For example, the two currently approved GE plant varieties from public institutions, papaya (Gonsalves, 1998; Swain and Powell, 2001) and flax (McHughen et al., 1997), started with only about 30 sister lines.
Unintended Effects from Genetic Engineering
Unexpected and unintended effects can be seen with all methods of breeding. Traditionally breeders observe such off-types regularly; they methodologically eliminate these individuals during the evaluation process, long before prepara-
tions are made for commercial release. An unexpected or unintended effect does not imply a health hazard, although clearly a plant expressing novel and unexpected characteristics warrants closer inspection prior to commercial release.
A large body of transgenic plant material is developed by university and government laboratories investigating aspects of rDNA that are not related to commercial interests. The Organization for Economic Cooperation and Development (OECD) lists more than 10,000 field trials with GE plants that were conducted between 1986 and 1999. Most of these authorized trials were conducted on plants genetically engineered by public universities and government research institutions, not for commercial release but to test the plants for unexpected or unintended results (see OECD, 2000). Consequently, most of the information on unexpected or unintended results comes from these sources.
Because GE crops are regulated to a greater degree than are conventionally bred, non-GE crops, it is more likely that traits with potentially hazardous characteristics will not pass early developmental phases. For the same reason, it is also more likely that unintentional, potentially hazardous changes will be noticed before commercialization either by the breeding institution or by governmental regulatory agencies.
The following are among the examples of unexpected or unintended characteristics that are often cited in the scientific literature.
Mycotoxin Content in Bt Corn
Bt (Bacillus thuringiensis) corn was developed to help farmers protect corn crops from insect pests, particularly the European corn borer and, more recently, the corn root worm. An unexpected effect in this product was a substantial reduction in mycotoxins, which can adversely affect animal and human health. Researchers hypothesized that this is because mycotoxin production is stimulated by fungal spores that infect corn when worms bore into and injure the plant. In the absence of insect damage (as in the Bt corn plants), there is reduced opportunity for pathogenic fungal spores to infect the plant and therefore less opportunity to generate the associated and undesirable mycotoxins (Munkvold et al., 1997, 1999).
Increased Lignin in Bt Corn
Saxena and Stotzky (2001) reported that three Bt corn varieties increased their stem lignin content relative to their respective non-Bt isogenic parents. Since the increase was found in more than a single variety, this suggests that the lignin increase is directly associated with the inserted DNA and is not simply an isolated coincidence. However, increased lignin content has not been recorded in other Bt corn lines, so it is uncertain whether the reported increase was due to the rDNA insertion, the presence of Bt endotoxin, or some other mechanism. Al-
though lignin is a normal component of plants and of the human diet, and the increased lignin content in the stems of these corn plants is not so great as to present a novel health hazard, the authors do suggest that there might be an environmental consequence from a corn plant with lignin content that is significantly higher than normal.
Petunias with Diminishing Color Over Generations
In this example, a GE petunia line was noticed to have a diminished flower color pattern and intensity over the course of several generations. Molecular analysis showed that a mutation occurred in a gene that regulates flower pigmentation and methylation was responsible for inactivating the gene (Meyer et al., 1992). This phenomenon occasionally occurs with conventional breeding, so its occurrence in a GE petunia does not indicate a unique GE phenomenon. This mutation appears to have occurred in only one GE petunia line out of hundreds generated and tested, thus it is not a general or systematic event.
Insertion of transfer DNA does not guarantee active expression of the associated gene. Inserted genes can be and are silenced or inactivated by the host plant by any of several mechanisms, including methylation (Dominguez et al., 2002). This is a well-known phenomenon and part of the reason genetic engineers generate as many different transgenic plants as possible.
Other Examples
Unexpected or unintentional effects, such as GE soybean stem splitting (Gertz et al., 1999) or Arabidopsis with unusual fertility or outcrossing characteristics (Bergelson et al., 1998), have been attributed to the rDNA breeding process.
The GE soybean stem-splitting case documented that a high proportion of GE soybeans suffered split stems in dry, hot conditions. Unfortunately, the non-GE parent line was not measured for stem splitting under similar conditions, but other non-GE soybean varieties were, revealing that a high proportion also suffered split stems. They were not as prone to splitting, however, as the indicated GE line, but that line was within the general accepted range for soybeans. Whether the GE soybean lines tested were more prone to splitting than their parents remains unknown, and thus conclusions cannot be drawn as to whether the genetic engineering process contributed to this trait.
In the Arabidopsis example, the authors compared the outcrossing of GE varieties with the outcrossing of a mutated strain that possibly had compromised fertility to begin with and was not an isogenic or near-isogenic line, necessary for properly conducting the type of experiment described.
Other unexpected effects in GE plants have been documented. In GE high oleic soybean lines, metabolic analysis revealed trace amounts of an unintended metabolite, cis9,cis15-octadecadienoic acid, an isomer of the fatty acid linoleic
acid that is not usually present in nonhydrogenated soybean oil, but is present in hydrogenated soybean oil and in other food sources. Because it is a component of other foods, it was not considered a health threat. The developer of the lines, citing Kitamura (1995), argued that the fatty acid substitution was actually nutritionally advantageous. After consideration, the Food and Drug Administration (FDA) determined that the presence of the unexpected linoleic acid isomer and glycinin did not pose a health hazard (DHHS, 1996). USDA also evaluated the GE soybeans, as it does all GE plants, and approved it (APHIS, 1997). FDA did determine that the high oleic soybeans were not substantially equivalent to regular soybeans due to the intended effect of an elevated oleic acid content, thus the breeder had to distinguish them from standard commodity soybeans.
The unexpected effects in these GE soybeans were small and required highly sensitive analytical tools to identify. Such small changes should be expected—although the exact metabolites and levels would remain unpredictable. Furthermore, because genetic changes result in protein changes, metabolic differences due to the presence or actions of new or differing levels of proteins cannot be unexpected. Such changes remain unintended, but they are common occurrences in new crop varieties, including new varieties from conventional breeding (although stringent and detailed analyses of conventional varieties are not conducted).
An important consideration is how these examples of apparent unpredictability with rDNA compare with similar unpredicted effects from conventional breeding methods. Unfortunately, the comparisons typically are made only between these unusual rDNA examples and, if conventional plants are compared at all, it is not with isogenic lines but with mutant lines or with less closely related commercial varieties. The correct comparison should be between commercial cultivars of rDNA derivation and isogenic parental varieties. For example, the comparison between Arabidopsis and a herbicide-tolerant variety is misleading because the comparator was a mutant variety of uncertain genetic composition. In addition, as discussed earlier, undesirable, unexpected, and unintended traits are noted in conventional breeding lines of commercial crop species on rare occasions, and these lines are consequently discarded.
ANIMAL BREEDING
Conventional Animal Breeding
Genetics is crucial for any livestock enterprise and requires breeders to evaluate production conditions and market objectives; the best breeds or lines of livestock for meeting market goals; selection of a breeding system and breed types; and selection of individual animals within the breed type. Generally, production conditions—so called gene-environment interactions—and market factors have the greatest influence on conventional animal breeding strategies.
Breeders develop a breeding plan that may either be continuous if replace-
BOX 3-3 Certain variables are used in a selection index to make decisions on which sires and dams to use in a breeding plan. Desirable traits include:
Additional traits of merit may include:
|
ment females are selected from within the herd or flock, or be terminal if all breeding females are selected and brought into the herd or flock. The breeding system may involve straight-breeding using only one breed, or cross-breeding using two or more breeds, with the latter expected to provide hybrid vigor, known as heterosis. In sound breeding programs, sires are chosen carefully as they have a great impact on production traits, and dams are chosen carefully based on expected high fertility and desired maternal traits (see Box 3-3). Selection of individual animals for breeding systems within herds or flocks is important, but most animal breeding plans depend on information called “expected progeny difference,” which is an estimate from across all animals within a breed of the genetic potential for desired traits an individual can transmit to progeny.
Unintended Effects from Conventional Animal Breeding
Double-Muscling
In beef cattle some non-GE mutant animals show the presence of extraordinary quantities of muscle. The muscular hypertrophy, referred to as double-muscling, is a heritable trait that primarily results from an increase in the number of muscle fibers or cells rather than an increase in the size of individual muscle fibers.
This phenotype has been observed in several cattle breeds. The breed in which this phenotype has been most studied is the Belgian Blue, which has been extensively and systematically selected for double muscling to the point that it is a heritable trait in many herds. Cattle with this phenotype are very lean and produce about 20 percent more lean edible meat (Hanset, 1986; Shahin and Berg, 1985).
Problems with stress tolerance, fertility, and calf viability may have unintended effects in exploiting the double-muscling phenotype for beef production. Researchers established that a genetic deletion in the myostatin gene, which codes for proteins in muscle, was the cause of double muscling in Belgian Blue cattle (Grobet et al. 1997). They also determined the entire coding sequence of the myostatin gene in 32 animals with extreme muscle development from 10 European cattle breeds and found 5 sequence polymorphisms that disrupted the function of the myostatin protein (Grobet et al., 1998).
Porcine Stress Syndrome
In pigs a condition referred to as porcine stress syndrome (PSS), or malignant hyperthermia, has been identified. Affected animals have a higher incidence of death from stress. Pigs with PSS that are harvested at packing plants have pork that is pale in color, exudes excessive water, and is soft in texture. Postmortem, pork from PSS pigs is referred to as PSE—pale, soft, and exudative. PSE pork has significant adverse meat quality attributes, and many consumers find the product objectionable. PSS is the result of intensive breeding of pigs to select animals with increased muscle mass, and it results from an unintended mutation in the ryanodine-receptor gene (Wendt et al., 2000).
Homozygous pigs are more susceptible to stress than are heterozygous pigs. A test that accurately identifies the genetic mutation in pigs that causes PSS has been developed and is widely used in selection programs. Use of this test has resulted in a reduced incidence of the mutation in breeding stock.
Biotechnology
Biotechnology will influence the development of conventional animal breeding. Importantly, those traits that breeders desire to include in their breeding plans are physiologically complex and controlled by multiple genes with variable effects. The overlay of biotechnology and conventional breeding will likely occur in phases that involve broad genetic maps with informative microsatellite markers and evolutionarily conserved gene markers; use of microsatellite markers to identify quantitative trait loci (QTL) of commercially important traits, based on knowledge of complex pedigrees or crosses between phenotypically and genetically divergent breeds or strains; identification of specific trait genes and/or use of conserved markers to identify candidate genes based on their position in gene-
rich species, breeds, or strains; and phenotype mapping through functional analysis of trait genes, linking the genome through physiology to the trait.
The use of biotechnology techniques, such as marker-assisted selection, is expected to increase among cattle and swine industries, whereas the poultry industry prefers quantitative genetics. The rigorous use of quantitative genetics in the poultry industry will likely continue as a result of the low cost per animal, short generation intervals, and the ability to address a complex array of traits. Nevertheless, it seems clear that desired complex traits will be understood through the use of genomics information and that the credibility of the technologies of marker assisted and QTL selection in animal breeding programs will be validated and used extensively.
Genetic Engineering
As with plant breeding, animal breeding programs and the production of transgenic farm animals can result in unintended effects. Most, but probably not all, gene-based modifications of animals for food production or therapeutic claims fall under the FDA Center for Veterinary Medicine regulations of new animal drugs. The animal drug provisions of the Federal Food, Drug, and Cosmetic Act provide the legal framework for developing science-based guidelines for assessing the commercial value of these products to society. Other products of transgenic methods will no doubt be developed that could be viewed as containing food or color additives and vaccines. Development of site-specific gene insertion techniques and animal genome projects could change the scope of potential genetic modifications to yield a wider variety of products than are currently being investigated. The following are some examples of unintended effects of recombinant technology administered to animals.
Recombinant Bovine Somatotropin
Commercial sales of recombinant-derived bovine somatotropin (bST) began in the United States during 1994, and its use has gradually increased such that approximately half of U.S. dairy herds, comprising more than 3 million cows, are receiving bST supplements (Bauman, 1999; Etherton and Bauman, 1998). The supplements, which are delivered through an injection of sustained-release formulation every 14 days, result in marked improvements in productive efficiency while maintaining normal cow health and herd life (Bauman, 1999; Etherton and Bauman, 1998). Supplements containing bST are being used commercially in 19 countries.
In animals treated with bST, the yield of milk is increased by 10 to 15 percent (approximately 4-6 kg/d), although even larger increases may occur when the management and care of the animals are excellent (Bauman, 1992; Chilliard, 1989; NRC, 1994). The pattern of response is one in which milk yield gradually increases over the first few days of bST treatment and reaches a maximum during
the first week. When treatment is continued, the increased milk yield is sustained. Thus bST results in a greater peak milk yield and an increased persistency in yield over the lactation cycle.
As a consequence of these changes in the lactation curve, commercial practice has shifted to an extended calving interval, which results in fewer births per herd, lower incidence of postpartum metabolic diseases, lower veterinary costs, and an overall improvement in herd life, animal well-being, and dairy farm profitability (Van Amburgh et al., 1997).
Recombinant Porcine Somatotropin
During the past 20 years much has been learned about how porcine somatotropin (pST) increases the growth of pigs (Etherton, 2000; Etherton and Bauman, 1998). These advances were facilitated by the development of methods to produce recombinant-derived pST on a large scale. The availability of large quantities of recombinant pST enabled landmark studies to be conducted that evaluated how the administration of pST affected muscle and adipose tissue growth. Administering pST by intramuscular injection to growing pigs can increase muscle growth by as much as 50 percent and, concurrently, decrease adipose tissue accretion with a maximal effect of about 70 percent. The results of pST administration are due to an array of biological effects from the hormone involving coordinated changes in lipid, protein, and carbohydrate metabolism (Etherton and Bauman, 1998; NRC, 1994). Currently approved for use in 14 countries, pST is undergoing testing required by FDA for commercial use in the United States.
There have been no reported unintended effects at doses of pST that are used in commercial swine production. There is, however, some evidence at much larger doses that pST can cause osteochondrosis, which is an abnormality characterized by an unmineralized, nonvascularized plug of cartilage in the metaphysis of the epiphyseal growth plate (Evock et al., 1988).
Unintended Effects from Genetic Engineering
The report Animal Biotechnology: Science-Based Concerns describes some examples of unintended effects found in transgenic pigs (NRC, 2002). The introduction of DNA into random sites in the genome is a mutagenic event that will affect any gene at or near the site of introduction, potentially resulting in unintended effects in the target animal. Given the remarkable variation in sites of gene insertion, the number of gene copies transferred, and the level of gene expression, every animal produced by microinjection is potentially unique in its phenotype (NRC, 2002). This variability contributes enormously to any effort to assess the unintended effects of genetic modification and to evaluate whether any unintended effect that occurs is possibly a cause for a health concern in humans.
An example of a detrimental effect resulting from overexpression of a transgene is illustrated by studies conducted by USDA with transgenic pigs. These studies were the logical extension of earlier work showing that daily injection of recombinant pST markedly increased growth rate, improved the ratio of muscle to fat, and improved feed efficiency (reviewed in Etherton, 2000; Etherton and Bauman, 1998). In these studies, transgenic pigs were created with a gene for human somatotropin or bST to test the hypothesis that overexpression of human somatotropin would result in effects comparable with those observed in studies in which pigs were injected with recombinant pST. Some of the transgenic pig lines did exhibit increased weight gain and were more efficient in converting feed to body weight gain; however, the pigs that showed high levels of either human somatotropin or bST also had a variety of physical problems, including lameness, lethargy, and gastric ulcers (Pursel et al., 1990).
Cloned Animals
A detailed discussion of animal cloning, including the committee’s findings and recommendations related to this area, can be found in the subreport included with this volume. The following is a brief overview of unintended effects associated with cloning technology.
Cloning by nuclear transfer from embryonic blastomeres (Willadsen, 1989; Willadsen and Polge, 1981) or from a differentiated cell of an adult (Kuhholzer and Prather, 2000; Polejaeva et al., 2000; Wilmut et al., 1997) requires that the introduced nucleus be reprogrammed by the cytoplasm of the egg and direct development of a new embryo, which is then transferred to a recipient mother to develop to term. The resulting offspring will be identical to their siblings and to the original donor animal in terms of their nuclear DNA, but will differ in their mitochondrial genes and possibly the manner in which the nuclear genes are expressed. Cloning from blastomeres and somatic cells may result in large calves and lambs, the so-called “large offspring syndrome” (Sinclair et al., 2000; Young et al., 1998). More serious abnormalities may also be associated with cloning from somatic cells (Wilmut et al., 1997).
Marker-Assisted Selection
The use of marker-assisted selection in both plants and animals is expected to increase exponentially as data from genome sequencing projects and the density of useful segregating markers increase for economically important species. Marker-assisted selection is already helping plant breeders to identify desirable individuals from heterogeneous populations and to segregate for disease resistance and other features that would ordinarily require several years and substantial field plot experiments. Animals in breeding programs initially will be screened for genes that control simple traits, such as horns, which are undesirable in cattle,
and halothane sensitivity, which segregates with metabolic stress syndrome in pigs. As this technique develops over time, easily identifiable markers will be chosen that accompany multiple genes that control more complex traits, such as meat tenderness and taste, growth, calf size, and disease resistance.
Although marker-assisted selection has enormous potential for improving animal health and production traits, it may decrease genetic diversity (Dekkers and Hospital, 2002). Thus short-term gains in productivity may occur at the expense of longer-term improvements due to the extended time before an unintended effect is identified (Dekkers and Hospital, 2002; Dekkers and Van Arendonk, 1998).
It is possible that marker-assisted selection in animal breeding is beneficial because there is a cumulative effect of expression of multiple genes that may be important, whereas effects of individual genes within the chromosomal region associated with the marker are too minor to be of economic importance. Thus the cumulative effects of multiple genes may be exploited to a greater extent by marker-assisted selection for a single gene with a major effect in an attempt to enhance a desired trait. Additionally, this type of marker-assisted selection may counter inbreeding by encouraging breeding strategies that maintain diversity among major genetic loci through exploitation of genes from rare breeds and wild ancestors to improve traits for disease and parasite resistance and adaptability.
MECHANISMS BY WHICH UNINTENDED EFFECTS IN GENETICALLY ENGINEERED ORGANISMS ARISE
Unintended effects may arise from one or more of several mechanisms, which can be either systemic or individual. Systemic effects are those likely to appear in all or almost all transgenic plants, animals, or microbes transformed using the same genetic engineering method and DNA construct. Individual effects, in contrast, are likely due to the nature of a particular transformation event in a single organism, occur in only one transgenic line, and are not displayed by other plants of the same species transformed using the same genetic engineering method. Consequently, individual events may provide greater opportunity to broaden understanding of otherwise unidentified mechanisms that may be responsible for unintended changes.
Some possible mechanisms that may result in unintended effects include the following.
-
The sequence of interrupted DNA may be a functional gene, resulting in a loss or gain of whatever function that gene provided.
-
Chromosomal changes may occur depending on the location of the insertion.
-
The host recipient may have an unusual genotype within the population being sampled that will be expressed and observed in the transgenic organism, an effect known as residual heterozygosity.
-
Somaclonal variation or spontaneous mutation may occur in the tissue culture phase of the transformation regeneration processes.
-
Interaction may occur between novel gene products and endogenous products—a systemic effect shown in most or all transformation events with the same gene.
When the unexpected effect is systemic and observed with most or all transgenic events, then the likely explanation resides with the function of the expressed introduced gene. For example, an enzyme introduced into a microbe may interact with new substrates, leading to new products in addition to or instead of intended products. That is, the inserted gene might generate the expected protein, but that novel protein might react with metabolites in the new host that differ from those in its native organism. This has occurred in conventional plant breeding when new metabolites, novel to both parents, were generated from crossing S. tuberosum and S. brevidens (Laurila et al., 1996). Kuiper and colleagues (2001) list several examples of documented unexpected effects in transgenic plants.
Genetic Instability
A frequently stated concern about the use of rDNA technology is that transgenic organisms may be genetically unstable. That is, due to the transfer of genetic material using rDNA methods, the host organism may have some as yet unknown and unobserved mechanism to identify and eliminate the intruding DNA. However, transgenic plants, animals, and microbes have been studied for long enough and intensively enough to determined that genetic instability is not a routine phenomenon. While it is known that transgenic organisms can, on occasion, silence the expression of inserted genes through mechanisms that are now well studied, this phenomenon occurs on an event-by-event basis—not to all transgenic organisms resulting from a particular method or a particular construct.
Although genetically unstable plant varieties can be produced, their likelihood for commercialization is very low. First, if the new trait is unstable, then the organism loses the new trait and reverts back to the traits inherited from the parent plant. Second, domestic and international plant protection regulations exist to protect the intellectual property rights of plant breeders (for more information, see the U.S. Plant Variety Protection Act [P.L. 91-577, 1970]; and the International Convention for the Protection of New Varieties of Plants [UPOV, 1961]). These regulations provide patent-like protection for new varieties of plants under a certificate program. Since only stable varieties are eligible for certification, their production will be favored over unstable varieties.
Gene Transfer Using Foreign DNA
Genetic engineering can be used to transfer DNA within species or across species. In considering examples of cross-species transfers, plants, animals, and microbes all have been engineered with DNA from distant species to give rise to new GE products with realized or potential commercial application. Most examples of commercial GE crops carry DNA from unrelated species, commonly bacteria.
While the process of crossing plant and bacterial DNA is a challenge for conventional plant breeders, gene transfer from bacteria to plants does occur in nature. An example is Agrobacterium (see Chapter 2), which has the capability of transferring a portion of its own DNA into plants, where it integrates into the plant genome. In most cases bacteria do not exchange DNA with plants or animals. Genetic engineering transfer of foreign DNA into animals, such as inserting a growth hormone gene into salmon to generate a faster-growing fish, is currently under review by FDA.
Many microbes have been engineered with genes from plants or animals. GE microbes are used to produce a wide range of pharmaceuticals. For example, bacteria are engineered with the human gene for insulin to produce recombinant human insulin. GE microbes are also used to produce products used in food processing. For example, GE bacteria produce the enzyme chymosin used in cheese making. This product was one of the first GE foods approved for use in the United States and in the United Kingdom (FDA, 2001; University of Reading, 2004).
Gene Transfer Using Same-Species DNA
GE foods have been identified as potentially hazardous because they carry genes from foreign species. However, combining genes from different species may or may not be hazardous, as all organisms, including humans, carry genes inserted from different species. For example, all humans carry genes that have been incorporated from viral infections (Cohen and Larsson, 1988).
Genetic engineering is one method that can be used to transfer genes from either related or unrelated species into a host genome. For example, the Xa21 gene is derived from the rice relative Oryza longistaminata. This gene confers the valuable agronomic trait of disease resistance, and so various rice varieties have been developed using both conventional crossing and rDNA technology to add the Xa21 gene to commercial rice varieties. Regardless of the method of breeding, from the standpoint of composition, the two new rice varieties are virtually indistinguishable.
Another example includes high oleic acid soybeans, discussed earlier. Both conventional breeding via induced mutagenesis and rDNA transfer of genes from other soybean varieties have been used to generate new soybeans with a higher
proportion of oleic acid. However, the conventionally bred high oleic soybeans can be unstable—depending on environmental conditions, their fatty acid content may vary—while the GE high oleic soybean remain more stable (DHHS, 1996).
Other rDNA examples exist where foreign DNA is not necessarily present. The first GE food product approved for human consumption in the United States, the Flavr Savr tomato, was engineered with an inverted tomato gene (CFSAN, 1994), along with bacterial genes transferred to facilitate selection of the modified plant. In the absence of foreign DNA or genes from another species, an argument may be made that a GE plant is not transgenic and not unnatural if only native DNA is transferred. One could readily transfer genetic material from one tomato variety to another, or rice to rice, or soybean to soybean without any other contributing source of DNA.
A recent report of a similar case involved a coffee variety being engineered to produce less caffeine as an alternative to current industrial methods of decaffeination (Ogita et al., 2003), which may use benzene or other organic solvents for extraction. Using rDNA methods, Ogita and colleagues (2003) were able to transform coffee and observe a 70 percent reduction in caffeine in leaves. Because their intent was to interfere with the natural caffeine biosynthetic pathway in the coffee plant, the objective also could have been pursued with an induced mutation breeding program. However, the rDNA method was preferred because it was more precise and predictable, as well as less likely to induce deleterious mutations unrelated to caffeine.
Naturally Occurring rDNA and Human-Mediated rDNA
Genetic recombination occurs in both nature and in human-mediated genetic engineering of plants, animals, and microorganisms (see Box 3-4). This section examines recombination as it occurs in nature and as it occurs with genetic engineering, with particular reference to differences between the two processes. Natural recombination occurs in several ways, typically divided between homologous and nonhomologous recombination events. Genetic recombination events that occur specifically in animals are summarized in Chapter 2 and are discussed in detail in the report Animal Biotechnology: Science-Based Concerns (NRC, 2002).
Homologous Recombination
Homologous recombination occurs when DNA strands with similar or homologous base sequences spontaneously recombine with one another. Such juxtaposed sequences do not have to be identical for recombination to occur, but the incidence of recombination between nonidentical sequences diminishes in proportion to the degree of sequence dissimilarity (Lewin, 1985).
Chiasmata are chromosomal crossover events, visible under the microscope, in which whole segments of chromosomes are exchanged. These exchanges often
BOX 3-4 Prior to the use of recombinant deoxyribonucleic acid (rDNA) techniques to achieve recombination of genes from different species, crossing among similar species occurred both with and without human intervention. An example of such naturally occurring “intergeneric” crossing is the wheat that arose thousands of years ago from the incorporation of genes from three different plant species. Many common varieties of modern bread-type wheat carry a fragment of a rye chromosome. When this intergeneric hybridization occurred, it provided the host wheat with a disease-resistance gene—along with an undetermined, uncharacterized, and unregulated number of other genes carried along on the same rye chromosome fragment—and consequently was adopted by plant breeders as a useful source for disease resistance in the wheat gene pool. Such naturally occurring gene transfers across species are frequent; for example, several genetic translocations between wheat and rye have occurred as a result of separate incidents involving commercial bread-type wheat. Not all species can exchange DNA without human intervention. Even in the case of closely related species, exchanges of genes occur at low frequency and depend on humans to identify and select the rare events to stabilize the genes and traits. Without such intervention, the resulting hybrids would likely die out or take evolutionary time spans to become established, as in the case of bread-type wheat. Human plant breeders have used their knowledge of interspecies gene transfer to create new species, employing techniques as simple as pollen to pistil transfer. Triticale is an example of an intergeneric hybrid between wheat (Triticum) and rye (Secale). Triticale was developed as a crop variety that combined the bread-making quality of wheat with the ability of rye to grow in harsh environments (Larter, 1995). Unexpectedly, initial results from this cross expressed the wrong combination that resulted in a weak plant with poor baking quality. Subsequent crosses produced Triticale hybrids that worked well enough to be grown with modest commercial success in various regions, including Europe, North America, and Australia. Today, Triticale is widely grown and used both in animal feeds and as an ingredient in multigrain breads. The potato is another example of a common food developed from a combination of genes from different species. Several popular potato varieties were developed using conventional breeding methods to bring useful genes from foreign species into the common potato variety S. tuberosum (Jansky and Rouse, 2000). Additionally, some Nicotiana species carry genes naturally transferred by the bacterium, Agrobacterium, through nonhomologous recombination (Aoki et al., 1994; Frundt et al., 1998). While these naturally occurring events are possible either with or without human intervention, genetic engineering allows for the introduction of a greater variety of novel genes into a host genome (see Figure 3-1). |
involve dozens or even hundreds of genes, giving rise to new chromosomal structures. Such naturally occurring events are crucial to conventional crossbreeding as they allow the breakage of chromosomally linked undesirable genes from useful genes and the recombination of desirable genes.
Nonhomologous Recombination
Nonhomologous recombination is observed in species from phage and bacteria to plants and animals. As noted in Chapter 2, transposable elements—also known as transposons—are well-characterized genetic segments with the ability to insert into nonhomologous DNA sequences and to copy themselves and move elsewhere. Results of transposon activity include stable integrations into the target DNA and loss of genetic function if the insertion interrupts a previously functional gene; complete excisions with restoration of function of previously interrupted gene; and rearrangements around the site of integration.
Nonhomologous recombination allows the DNA to be inserted into dissimilar sequences, which means almost anywhere in the genome. Such latitude allows the possibility of insertion into a functional gene, thus inactivating it, in contrast to homologous recombination where the DNA sequence at the locus of insertion is known, that is, identical or near identical with the insertional DNA with the recombined gene generally retaining functionality. This factor makes nonhomologous recombination less attractive to breeders than homologous recombination because nonhomologous recombination events will have to be more stringently screened to identify and eliminate inserts into and possible inactivation of nontarget genes.
In practice, insertion of transferred DNA, or T-DNA, into crucial genes is a relatively rare event in crop species and in application to crop improvement. The standard breeding practices of candidate variety evaluation and screening to eliminate lines showing undesirable changes identifies and eliminates those lines with inserts that inactivate important genes. Genetic engineering also has the distinct advantage over non-genetic engineering methods because the sequence of the inserted DNA is known, and it can be used to identify the precise location of the insert in the genome and characterize nearby genes.
Preferential Insert Loci
Recombinational “hotspots” are hypothetical locations in the genome where T-DNA is preferentially inserted. The implication is that these targeted loci, for which sound evidence in plants is lacking, are regions of unstable DNA so any insert would be similarly unstable. It seems logical to predict, then, that if an unstable segment of DNA is inserted into an unstable sequence of host DNA, then the combined instability would lead to unstable, unintended results.
This interesting conjecture remained unanswered for years and was the basis
of considerable debate in the Agrobacterium transformation community. Recent analyses of Agrobacterium T-DNA inserts into Arabidopsis provide data to address the question. The Salk Institute maintains a population of 32,500 Arabidopsis thaliana lines with Agrobacterium-mediated T-DNA inserts in and around the 25,500 genes in the genome of this species. An analysis shows the loci of insertion to be reasonably evenly distributed along the entire genome. Some of the inserts are between genes and others interrupt genes (Ecker, 2003).
In a related study comparing T-DNA with transposons inserted into the Arabidopsis genome, for transposons a slight preference was found for insertion into coding sequences of genes. As with the study of Ecker (2003), Pan and colleagues (2003) found that both T-DNA and transposon insertions are reasonably evenly distributed throughout the genome.
These data indicate that there is no strong recombinational hotspot or strong preferential insert site for T-DNA. Similarly, there is no evidence to suggest the CaMV 35s promoter in GE plants is any more unstable than the CaMV 35s promoter in ordinary plants infected with CaMV.
Recombination Hotspots and Genetic Instability
The range of loci in the Arabidopsis genome into which T-DNA has been inserted indicates that the insertion location is random or near random (Ecker, 2003). If insertional hotspots do exist, they clearly are not exclusive, but at most they are mildly preferred points of recombinational insertion. Genetic instability at singular loci in itself is not hazardous unless the unstable nature necessarily gives rise to hazardous outcomes. However, genetic instability, when it occurs, affects only one cell or plant at a time, and the result is typically a loss of activity of the relevant gene. An example is the case where a herbicide tolerance gene is inserted into an unstable locus and then grown in a field of the herbicide-tolerant plants. When an instability incident occurs in this crop, the herbicide tolerance is lost—the plants experiencing this instability event revert to being herbicide susceptible. When the field is then sprayed with the herbicide, only those few individual plants suffering the instability succumb to the spray.
Because the timing of such destabilizing events is rare and does not strike an entire population simultaneously, it is unlikely that the loss of a small number of crop plants would be noticed compared with the loss of weeds or with nonherbicide-tolerant volunteer crop plants. If genetic instability were more common or synchronized, such that it were noticeable, the breeder would not be able to obtain certification for the new variety due to failure to meet the genetic stability requirement.
Genetic instability is observed from time to time, both in conventional breeding and in genetic engineering. Robertson (1978) described a conventional corn line with a high degree of genetic instability due to an endogenous transposon. As
discussed earlier, unstable pigmentation in GE petunias provides an example of a systemic instability in particular GE plants (Meyer et al., 1992).
Spontaneous mutations change DNA also, affecting everything from single base changes, known as “point mutations,” to entire genomes. Deletions of entire segments from a genome are predictably severe and deleterious, but point mutations or even more substantial mutations, such as those affecting large tracts of DNA in chromosomes, can be beneficial and adaptive.
Because of the ubiquitous nature of spontaneous mutations, all plant varieties—whether conventional or GE—exhibit a small degree of genetic instability and generate mutants with some degree of frequency. If the frequency of spontaneous genetic instability is low, the typical loss-of-function mutants will probably not be noticed; if it is high, the variety cannot be commercialized.
Natural types of recombination can also result in the same effects. Transposons, even Agrobacterium insertions, all interrupt any DNA sequence where they insert, regardless of whether they insert into homologous or nonhomologous DNA. Depending on the function of the interrupted DNA, there may or may not be phenotypic consequences from the insertion.
Agrobacterium naturally inserts DNA into the host plant-cell genome, typically in a genetically stable manner. The substitution of desirable DNA by genetic engineering for the phyto-oncogenic DNA of the wild strains does not affect the mechanics of transfer. The genes responsible for Agrobacterium genetic transformation and recombination in the plant are physically and functionally separate from the T-DNA actually transferred and integrated. The mechanics of Agrobacterium appear the same whether the event is staged through a natural infection process by a crown gall-producing wild-type strain or by the same strain that has been disarmed by the removal of the gall-producing genes and subsequently replaced with known, desirable DNA.
THE GENETIC MANIPULATION CONTINUUM
Overview
Predicting the likelihood of unintended hazards from compositional changes associated with genetic modifications does not fit a simple dichotomy comparing genetic engineering with non-genetic engineering breeding. This is because there are many mechanisms shared in common by both GE and non-GE methods, and also because there are techniques that slightly overlap each other. Furthermore, within the scope of genetic engineering (rDNA) technology, several mechanisms for genetically transforming plants are available as options to scientists, such as Agrobacterium-mediated gene transfer and the use of the particle gun (McHughen, 2000). These two examples of genetic engineering are as different mechanistically as the conventional methods of narrow crossing and wide crossing (discussed in Chapter 2). Consequently, it is unlikely that all methods of
either genetic engineering or conventional breeding will have equal probability of resulting in unintended changes. It is the final product of a given modification, rather than the modification method or process itself, that is more likely to result in an unintended adverse health effect. In Figure 3-1 some of the methods used to generate GM plants are shown to illustrate the full range of possibilities that might lead to unintended changes (these methods are described in Chapter 2).
Analysis of the Continuum
As noted earlier in this chapter, unintentional changes are possible with all conventional and biotechnological breeding methods for genetic modification. It is possible to represent the likelihood of such changes as a continuum, albeit only a partially understood one. Placement along this continuum has no bearing on risk of adverse outcomes, but only on the probability of unintended changes, which need not be hazardous. The potential for hazard resides in specific products of the modification regardless of whether the modification was intentional or unintentional.
Again, unintended effects do not necessarily imply hazard. If a particular method were inherently hazardous, all products resulting from its use would be potentially harmful. However, it is known that each method can provide safe products, so the key for breeders and regulatory agencies, in their reviews of specific products, is to identify the relatively rare, potentially hazardous products resulting from any method.
In breeding, the developer can generate literally thousands of breeding lines; each might be progeny of the same parents, thus bringing together the same set of genetic information, and yet possess subtle differences. As noted previously in this chapter, conventional breeders may scrutinize 2,000 of these “sister lines” of a single cross of two parent plants. Meiotic recombination allows sister lines to show a continuum of phenotypic expression. Over several years, the breeder eliminates most, or sometimes all, of those breeding lines because they are unsuitable.
Plant breeding is often said to be a process not of selection, but of elimination. Any off-types, unstable lines, or lines showing characteristics such as significant differences in nutrient content, responses to environmental stresses, diseases, or the presence of other undesirable traits are discarded as soon as they are noticed. This winnowing takes place over several years, so the remaining lines identified for prospective commercial release are unlikely, but not guaranteed, to have any significant compositional changes other than those related to the desired trait. For this reason, regulatory scrutiny focuses most often on the new trait and its metabolic perturbations. Nevertheless, the appearance of subtle or obscure phenotypic changes can go unnoticed by breeders or regulators and may subsequently have to be recalled from the market, as in the case of the Lenape potato—
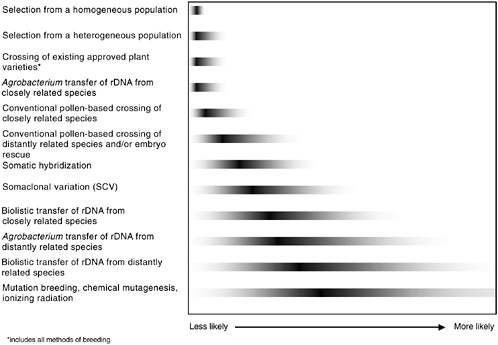
FIGURE 3-1 Relative likelihood of unintended genetic effects associated with various methods of plant genetic modification. The gray tails indicate the committee’s conclusions about the relative degree of the range of potential unintended changes; the dark bars indicate the relative degree of genetic disruption for each method. It is unlikely that all methods of either genetic engineering, genetic modification, or conventional breeding will have equal probability of resulting in unintended changes. Therefore, it is the final product of a given modification, rather than the modification method or process, that is more likely to result in an unintended adverse effect. For example, of the methods shown, a selection from a homogenous population is least likely to express unintended effects, and the range of those that do appear is quite limited. In contrast, induced mutagenesis is the most genetically disruptive and, consequently, most likely to display unintended effects from the widest potential range of phenotypic effects.
developed using non-genetic engineering methods—discussed previously in this chapter (Laurila et al., 1996).
The products of a narrow cross-pollination, from a plant of a given species to another plant of the same species, or even the same subspecies, are less likely, but not guaranteed, to pose unintended risks. This is due to the expectation that pollination is inherently safer than other methods of gene transfer because the range of resulting products is typically limited to those products already present in the species or subspecies. However, even this likely “safe” method is subject to occasional spontaneous mutations or infelicitous genetic recombination events leading to novel and unexpected products, and these products may carry some degree of risk, such as disease susceptibility, leading to, for example, hazardous levels of fungal mycotoxins. Expanding the range of conventional breeding beyond the same species provides a greater opportunity to introduce novel genetic information and thereby novel sources for unexpected effects.
Triticale is a man-made crop developed by breeding wheat with rye; hordecale is a similar product of barley and rye. Both examples bring together thousands of genes in a way that does not occur in nature and which conceivably could result in the production of undesirable toxicants. As discussed previously in this chapter, the conventional potato breeding program combining Solanum tuberosum and S. brevidens produced not only the expected toxicants, but also a new one, demissidine, which is not produced in either parent (Laurila et al., 1996).
Although some products can be reasonably predicted to be hazardous, this does not imply that all products of rDNA—or other methods of bringing together genes—are necessarily hazardous. Agrobacterium and the particle gun are the only two rDNA methods represented on the continuum in Figure 3-1 because they provide almost all of the GE crops approved for commercial release. They are depicted separately because of the documented predilection of the particle gun to introduce multiple, broken, or rearranged DNA segments, in contrast with the usually singular and high-fidelity transfer typical of Agrobacterium.
The more disorganized particle gun mechanism increases the risk of insertion into crucial endogenous sequences. The degree of increased risk is as yet undetermined and may be minimal. However, in both cases sufficient numbers of events are generated and screened to make it possible to identify those with the most desirable insertion features, the best expression of desired genes, and the least likelihood of deleterious features, in much the same way that conventional breeders evaluate their breeding lines.
Continuum Conclusions
Unintended adverse health effects can be either predictable or unpredictable. All organisms undergo spontaneous mutations, giving rise to novel traits, which may carry some hazard. The incidence of such mutations is relatively rare and the type of hazard associated with them is generally not predictable. On the other hand, introduced mutations, such as by rDNA, theoretically allow a gene from any species to be inserted into and expressed by a food crop. Clearly, such a product has the potential to be hazardous if the inserted gene results in the production of a hazardous substance. Although the process of rDNA is itself not inherently hazardous, the resulting product of the process may be.
DISCUSSION
This chapter reviews examples of unintentional changes that resulted from the genetic modification of various organisms intended for food and the likelihood of unintentional changes arising from multiple methods of genetic modification. All forms of genetic modification, conventional and modern, may potentially lead to unintended changes in composition, some of which may have adverse health effects.
The range of biotechnology methods, including genetic engineering, makes it possible to alter, add, or remove genes from conventional food organisms. This manipulation of genetic information may pose either risks or benefits to health or the environment. Examples abound in which a single gene can have a dramatic effect or no effect at all, depending on its features. Similarly, chromosomal changes can also have either a dramatic or no apparent effect, again depending on the features of the genes involved (NRC, 2002).
Risks to human health from genetic changes in foods must, therefore, be placed in proper context. The introduction of allergenic proteins is a potential adverse health effect of concern that could arise from genetic modification, including genetic engineering, of food. These methods of breeding, however, are not the only way that potential allergens are introduced into the food supply (see Chapter 5).
Although genetic modification techniques may introduce unpredicted adverse health effects, a given technique itself is not a determinant of many more com-
mon adverse effects, such as those that result from personal food intolerances or sensitivities; crops growing in soils containing toxicants; contamination from postharvest infection with pathogens; and adulterants inadvertently mixed into food. By comparison, genetic modifications to food account for a lesser likelihood for adverse health effects.
Most of the novel features in commercially available GE products focus on single genes, such as herbicide tolerance or pest resistance in crops. Products developed using biotechnology express a wide range of new features, some of which may be benign, while others, such as industrial or pharmaceutical products, may pose a greater threat to food safety. Conventional breeding methods have also provided new crop varieties with enhanced herbicide tolerance, pest resistance, and other non-nutrient characteristics, as well as enhanced nutritional profiles, posing potential risks from ingestion that are similar to genetic engineering. Similar comparative examples can be found in animal breeding and in microbial strains, in which conventional methods have produced new products with unintended hazards similar to those that may result as a consequence of the application of rDNA technology.
REFERENCES
Akeley RV, Mills WR, Cunningham CE, Watts J. 1968. Lenape: A new potato variety high in solids and chipping quality. Am Potato J 45:142-145.
Ames BN, Gold LS. 1999. Pollution, pesticides and cancer misconceptions. Pp. 18-39 in Fearing Food, J. Morris and R. Bate, eds. Oxford, UK: Butterworth Heinemann.
Aoki S, Kawaoka A, Sekine M, Ichikawa T, Fujita T, Shinmyo A, Syono K. 1994. Sequence of the cellular T-DNA in the untransformed genome of Nicotiana glauca that is homologous to ORFs 13 and 14 of the Ri plasmid and analysis of its expression in genetic tumors of N. glauca × N. langsdorfi. Mol Gen Genet 243:706–710.
APHIS (Animal and Plant Health Inspection Service). 1997. DuPont Petition 97-008-01p for Determination of Nonregulated Status for Transgenic High Oleic Acid Soybean Sublines G94-1, G94-19, and G-168. Environmental Assessment and Finding of No Significant Impact. Online. U.S. Department of Agriculture. Online. Available at http://www.aphis.usda.gov/brs/dec_docs/9700801p_ea.HTM. Accessed December 12, 2002.
Bauman DE. 1992. Bovine somatotropin: Review of an emerging animal technology. J Dairy Sci 75:3432–3451.
Bauman DE. 1999. Bovine somatotropin and lactation: From basic science to commercial application. Domest Anim Endocrinol 17:101–116.
Beier RC. 1990. Natural pesticides and bioactive components in foods. Rev Environ Contam Toxicol 113:47-137.
Beier RC, Oertli EH. 1983. Psoralen and other linear furocoumarins as phytoalexins in celery. Phytochemistry 22:2595–2597.
Bergelson J, Purrington CB, Wichmann G. 1998. Promiscuity in transgenic plants. Nature 395:25.
Berkley SF, Hightower AW, Beier RC, Fleming DW, Brokopp CD, Ivie GW, Broome CV. 1986. Dermatitis in grocery workers associated with high natural concentrations of furanocoumarins in celery. Ann Intern Med 105:351-355.
Birmingham, DJ, Key MM, Tubich GE, Perone VB. 1961. Phototoxic bullae among celery harvesters. Arch Dermatol 83:127-41.
CDC (Centers for Disease Control and Prevention). 1985. Phytophotodermatitis among grocery workers. Morbidity and Mortality Weekly Report 34(1):11–13. Online. Available at http://www.cdc.gov/mmwr/preview/mmwrhtml/00000464.htm. Accessed August 26, 2003.
CFIA (Canadian Food Inspection Agency). 2003. Seeds Act (R.S. 1985, c. S-8 ): Seeds Regulations. Updated to April 30, 2003. Online. Department of Justice, Canada. Available at http://laws.justice.gc.ca./en/S-8/C.R.C.-c.1400/172987.html. Accessed August 21, 2003.
CFSAN (Center for Food Safety and Applied Nutrition). 1994. FDA Backgrounder: Biotechnology of Food. Online. Food and Drug Administration. Online. Available at http://www.cfsan.fda.gov/~lrd/biotechn.html. Accessed December 12, 2002.
Chilliard Y. 1989. Long-term effects of recombinant bovine somatotropin (rBST) on dairy cow performances: A review. In: Sejrsen K, Vestergaard M, Neimann-Sorensen A, eds. Use of Somatotropin in Livestock Production. New York: Elsevier Applied Science. Pp. 61–87.
Cohen M, Larsson E. 1988. Human endogenous retroviruses. Bioessays 9:191–196.
Concon J. 1988. Food toxicology: Principles and concepts. Part A. New York, N.Y.: Marcel Dekker, Inc.
Dekkers JC, Hospital F. 2002. The use of molecular genetics in the improvement of agricultural populations. Nat Rev Genet 3:22–32.
Dekkers JCM, van Arendonk JAM. 1998. Optimum selection for quantitative traits with information on an identified locus in outbred populations. Genet Res 71:257–275.
DHHS (U.S. Department of Health and Human Services). 1996. High Oleic Acid Transgenic Soybean. Online. Memorandum of Conference. Online. Available at http://www.cfsan.fda.gov/~acrobat2/bnfM039.pdf. Accessed November 14, 2002.
Diawara MM, Kulkosky PJ. 2003. Reproductive toxicity of the psoralens. Pediatr Pathol Mol Med 22(3): 247–259.
Diawara MM, Trumble JT, Quiros CF. 1993. Linear furanocoumarins of three celery breeding lines: implications for integrated pest management. J Agric Food Chem 41:819-824.
Diawara MM, Trumble JT, Quiros CF, Hansen R. 1995. Implications of distribution of linear furanocoumarins within celery. J Agric Food Chem 43:723–727.
Dominguez A, Fagoaga C, Navarro L, Moreno P, Pena L. 2002. Regeneration of transgenic citrus plants under nonselective conditions results in high-frequency recovery of plants with silenced transgenes. Mol Genet Genomics 267:544–546.
Ecker J. 2003. A Sequence-Indexed Library of Insertion Mutations in the Arabidopsis Genome. Online. Salk Institute Genomic Analysis Laboratory. Available at http://signal.salk.edu/tabout.html. Accessed October 14, 2003.
Etherton TD. 2000. The biology of somatotropin in adipose tissue growth and nutrient partitioning. J Nutr 130:2623–2625.
Etherton TD, Bauman DE. 1998. The biology of somatotropin in growth and lactation of domestic animals. Physiol Rev 78:745–761.
Evock CM, Etherton TD, Chung CS, Ivy RE. 1988. Pituitary porcine growth hormone (pGH) and a recombinant pGH analog stimulate pig growth performance in a similar manner. J Anim Sci 66:1928–1941.
FAO/IAEA (Food and Agriculture Organization of the United Nations/International Atomic Energy Agency). 2001. FAO/IAEA Mutant Varieties Database. Online. Available at http://wwwinfocris.iaea.org/MVD/. Accessed January 1, 2003.
Finkelstein A, Afek U, Gross E, Aharoni N, Rosenberg L, Halevy S. 1994. An outbreak of phytophotodermatitis due to celery. Int J Dermatol 33:116-118.
FDA (U.S. Food and Drug Administration). 2001. Partial list of microorganisms and microbial-derived ingredients that are used in foods. Washington, DC: U.S. Food and Drug Administration, Center for Food Safety and Applied Nutrition. Available at http://vm.cfsan.fda.gov/~dms/opamicr.html. Online. Accessed December 3, 2003.
Frundt C, Meyer AD, Ichikawa T, Meins F. 1998. A tobacco homologue of the Ri-plasmid orf13 gene causes cell proliferation in carrot root discs. Mol Gen Genet 259:559–568.
Gertz JM, Vencill WK, Hill NS. 1999. Tolerance of transgenic soybean (Glycine max) to heat stress. In: Proceedings of the 1999 Brighton Conference—Weeds. Farnham, UK: British Crop Protection Council. Pp. 835–840.
Gilbert JC, Mohankumaran N. 1969. High tomatine tomato breeding lines. Veg Impr Newslett 11:6.
Gonsalves D. 1998. Control of papaya ringspot virus in papaya: A case study. Annu Rev Phytopathol 36:415–437.
Grobet L, Royo Martin LJ, Poncelet D, Pirottin D, Brouwers B, Riquet J, Schoerberlein A, Dunner S, Menissier F, Massabanda J, Fries R, Hanset R, Georges M. 1997. A deletion in the myostatin gene causes double-muscling in cattle. Nat Genet 17:71–74.
Grobet L, Poncelet D, Royo LJ, Brouwers B, Pirottin D, Michaux C, Menissier F, Zanotti M, Dunner S, Georges M. 1998. Molecular definition of an allelic series of mutations disrupting the myostatin function and causing double-muscling in cattle. Mamm Genom 9:210–213.
Hanset R. 1986. Double muscling in cattle. In: Smith C, King JW, McKay JW, eds. Exploiting New Technologies in Animal Breeding: Genetic Development. Oxford: Oxford University Press. Pp. 71–80.
Hellanäs KE, Branzel C, Johnsson H, Slanina P. 1995. High levels of glycoalkaloids in the established Swedish potato variety Magnum Bonum. J Sci Food Agric 68: 249-255.
Jansky SH, Rouse DI. 2000. Identification of potato interspecific hybrids resistant to Verticillium wilt and determination of criteria for resistance assessment. Potato Res 43:239–251.
Kitamura K. 1995. Genetic improvement of nutritional and food processing quality in soybean. JarqJpn Agr Res Q 29:1–8.
Kozukue N, Misoo S, Yamada T, Kamijima O, Friedman M. 1999. Inheritance of morphological characters and glycoalkaloids in potatoes of somatic hybrids between dihaploid Solanum acaule and tetraploid Solanum tuberosum. J Agric Food Chem 47(10):4478-4483.
Kuhholzer B, Prather RS. 2000. Advances in livestock nuclear transfer. Proc Soc Exp Biol Med 224:240–245.
Kuiper HA. 2003. Biotechnology, the environment, and sustainability. Nutr Rev 61(6, Supplement 1):105-109.
Kuiper HA, Kleter GA, Noteborn HP, Kok EJ. 2001. Assessment of the food safety issues related to genetically modified foods. Plant J 27:503–528.
Larter EN. 1995. Triticale. In: Slinkard AE, Knott DR, eds. Harvest of Gold. The History of Field Crop Breeding in Canada. Saskatoon: University of Saskatchewan. P. 367.
Laurila J, Laakso I, Valkonen JPT, Hiltunen R, Pehu E. 1996. Formation of parental type and novel alkaloids in somatic hybrids between Solanum brevidens and S. tuberosum. Plant Sci 118:145–155.
Leonardi C, Ambrosino P, Esposito F, Fogliano V. 2000. Antioxidative activity and carotenoid and tomatine contents in different typologies of fresh consumption tomatoes. J Agric Food Chem 48:4723-4727.
Lewin B. 1985. Genes II. New York: John Wiley & Sons.
McCue KF, Allen PV, Rockhold DR, Maccree MM, Belknap WR, Shephard LVT, Davies H, Joyce P, Corsini DL, Moehs CP. 2003. Reduction of total steroidal glycoalkaloids in potato tubers using antisense constructs of a gene encoding a solanidine glucosyl transferase. Acta Hortic 619:77-86.
McHughen A. 2000. Pandora’s Picnic Basket: The Potential and Hazards of Genetically Modified Food . New York: Oxford University Press.
McHughen A, Rowland GG, Holm FA, Bhatty RS, Kenaschuk EO. 1997. CDC Triffid transgenic flax. Can J Plant Sci 77:641–643.
Meyer PF, Linn F, Heidmann I, Meyer H, Niedenhof I, Saedler H. 1992. Endogenous and environmental factors influence 35s promoter methylation of a maize A1 construct in transgenic petunia and its color phenotype. Mol Gen Genet 231:345–352.
Moehs CP, Allen PV, Friedman M, and Belknap WR. 1997. Cloning and expression of solanidine UDP-glucose glucosyltransferase from potato. Plant J 11:227-236.
Munkvold GP, Hellmich RL, Showers WB.1997. Reduced Fusarium ear rot and symptomless infection in kernel of maize genetically engineered for European corn borer resistance. Phyto-pathology 87:1071–1077.
Munkvold GP, Hellmich RL, Rice LG. 1999. Comparison of fumonisin concentrations in kernels of trangenic Bt maize hybrids and nontransgenic hybrids. Plant Dis 83:130–138.
New Zealand Dermatological Society. 2002. Patient information: PUVA. Online. Available at http://www.dermnetnz.org/index.html. Accessed August 26, 2003.
NRC (National Research Council). 1987. Introduction of Recombinant DNA-Engineered Organisms into the Environment: Key Issues. Washington, DC: National Academy Press.
NRC. 1994. Metabolic Modifiers: Effects on the Nutrient Requirements of Food-Producing Animals. Washington, DC: National Academy Press.
NRC. 2000. Genetically Modified Pest-Protected Plants: Science and Regulation. Washington, DC: National Academy Press.
NRC. 2002. Animal Biotechnology: Science-Based Concerns. Washington, DC: The National Academies Press.
Ogita S, Hirotaka U, Yamaguchi Y, Koizumi N, Sano H. 2003. RNA interference: Producing decaffeinated coffee plants. Nature 423:823.
OECD (Organization for Economic Cooperation and Development). 2000. BIOBIN: A co-operative resource on safety in biotechnology, developed between OECD’s BioTrack Online and UNIDO’s BINAS. Online. Available at http://www1.oecd.org/ehs/biobin/. Accessed October 5, 2003.
Pan X, Liu H, Clarke J, Jones J, Bevan M, Stein L. 2003. ATIDB: Arabidopsis thaliana insertion database. Nucleic Acids Res 31:1245–1251.
Pastorello EA, Conti A, Pravettoni V, Farioli L, Rivolta F, Ansaloni R, Ispano M, Incorvaia C, Giuffrida MG, Ortolani C. 1998. Identification of actinidin as the major allergen of kiwi fruit. J Allergy Clin Immunol 101:531-537.
Polejaeva IA, Chen SH, Vaught TD, Page RL, Mullins J, Ball S, Dai Y, Boone J, Walker S, Ayares DL, Colman A, Campbell KH. 2000. Cloned pigs produced by nuclear transfer from adult somatic cells. Nature 407:86–90.
P.L. (Public Law) 91-577. 1970. Plant Variety Protection Act, 84 Stat. 1542—1559. Online. Available at www.ams.usda.gov/science/PVPO/PVPO_Act/whole.pdf. Accessed April 11, 2003.
Pursel VG, Hammer RE, Bolt DJ, Palmiter RD, Brinster RL. 1990. Integration, expression and germline transmission of growth related genes in pigs. J Reprod Fertil Suppl 41:77–87.
Robertson DS. 1978. Characterization of a mutator system in maize. Mutat Res 51:21–28.
Rowland GG, McHughen A, Bhatty RS. 1989. Andro flax. Can J Plant Sci 69:911–913.
Rowland GG, McHughen AG, Hormis YA, Rashid KY. 2002. CDC Normandy flax. Can J Plant Sci 82:425–426.
Sanford LL, Kowalski SP, Ronning, CM, Deahl KL. 1998. Leptines and other glycoalkaloids in tetraploid Solanum tuberosum × Solanum chacoense F2 hybrid and backcross families. Am J Potato Res 75:167-172.
Saxena D, Stotzky G. 2001. Bt corn has a higher lignin content than non-Bt corn. Am J Bot 88:1704–1706.
Shahin KA, Berg RT. 1985. Growth patterns of muscle, fat, and bone, and carcass composition of double muscled and normal cattle. Can J Anim Sci 65:279–293.
Sinclair KD, Young LE, Wilmut I, McEvoy TG. 2000. In-utero overgrowth in ruminants following embryo culture: Lessons from mice and a warning to men. Human Reprod 15:68S–86S.
Sinden SL, Webb RE. 1972. Effect of variety and location on the glycoalkaloid content of potatoes. Am Potato J 49:334-338.
Swain S, Powell DA. 2001. Papaya Ringpot Virus-Resistant Papaya: A Case Study. Online. University of Guelph. Available at http://www.foodsafetynetwork.ca/gmo/papayarep.htm. Accessed October 10, 2002.
University of Reading. 2004. Genetically modified food case studies: Chymosin. Reading, UK: University of Reading, National Centre for Biotechnology Education. Online. Available at http://www.ncbe.reading.ac.uk/NCBE/GMFOOD/chymosin.html. Accessed January 3, 2004.
UPOV (International Convention for the Protection of New Varieties of Plants). 1961. International Convention for the Protection of New Varieties of Plants, adopted by the Diplomatic Conference on December 1, 1961. Online. Available at http://www.upov.int/en/publications/conventions/1961/content.htm. Accessed May 22, 2003.
USDA-AMS (U.S. Department of Agriculture-Agricultural Marketing Service). 2001. The National Organic Program. Washington, DC: U.S. Department of Agriculture-Agricultural Marketing Service. Online. Available at http://www.ams.usda.gov/nop/indexIE.htm. Accessed June 14, 2003.
Van Amburgh ME, Galton DM, Bauman DE, Everett RW. 1997. Management and economics of extended calving intervals with use of bST. Livest Prod Sci 50:15–28.
Wendt M, Bickhardt K, Herzog A, Fischer A, Martens H, Richter T. 2000. Porcine stress syndrome and PSE meat: Clinical symptoms, pathogenesis, etiology and animal rights aspects. Berl Munch Tierarztl Wochenschr 113:173–190.
WHO (World Health Organization). 2000. Safety Aspects of Genetically Modified Foods of Plant Origin. Report of a joint FAO/WHO Expert Consultation. Geneva: WHO.
Willadsen SM. 1989. Cloning of sheep and cow embryos. Genome 31:956–962.
Willadsen SM, Polge C. 1991. Attempts to produce monozygotic quadruplets in cattle by blastomere separation. Vet Rec 108:211–213.
Wilmut I, Schnieke AE, McWhir J, Kind AJ, Campbell KH. 1997. Viable offspring derived from fetal and adult mammalian cells. Nature 385:810–813.
Young LE, Sinclair KD, Wilmut I. 1998. Large offspring syndrome in cattle and sheep. Rev Reprod 3:155–163.
Zimnoch-Guzowska E, Marczewski W, Lebecka R, Flis B, Schafer-Pregl R, Salamini F, Gebhardt C. 2000. QTL analysis of new sources of resistance to Erwinia carotovora ssp. atroseptica in potato done by AFLP, RFLP, and resistance-gene-like markers. Crop Sci, 40:1156-1167.
Zitnak A, Johnston GR. 1970. Glycoalkaloid content of B5141-6 potatoes. Am Potato J, 47: 256-260