1
Rationale for Military Interest and Current Capabilities in Monitoring Metabolism
Formidable monitoring capabilities exist for military hardware systems, but there is a lack of real-time information on the status of combat military personnel. Enhancing knowledge in this area would serve the U.S. Department of Defense (DOD) priority to assure the readiness of the Armed Forces (DOD, 2001). Based on the premise that metabolic processes are the bases of responses that allow organisms to survive in the face of environmental challenges and, thus, would be the earliest indicators of a change in physiological status, an understanding of regulatory mechanisms can suggest promising predictive markers of status and impending failure of adaptive response capabilities. Monitoring metabolic processes is needed to predict the readiness status of individuals in training and operational settings where human performance is important.
NEED FOR PHYSIOLOGICAL MONITORING
Monitoring combat service member status has become increasingly important as a result of new complex and lethal technologies that require high levels of cognitive readiness, such as computerized weapon systems; complicated communications and targeting devices; high-performance aircraft, tanks, and maritime vessels; and even the technologically advanced diagnostic systems used in the maintenance of military equipment. In addition, monitoring is necessary to ensure that operational personnel are as physically fit as possible because success on the battlefield is to a great extent dependent on the ability of combat service members to carry and operate weapons, to overcome physical obstacles, to traverse distances in harsh environments, and to endure a host of physical stresses and strains that could easily overwhelm unfit individuals. Also, battlefield tactics reduce line-of-sight contact with team members and increase the members’ geographical distance and isolation.
Physiological monitoring is not just a nice-to-have technological replacement for common sense or for good leadership (which includes understanding the signs of an individual combat service member’s limits). Combat service
members may not be aware that they are reaching dangerous levels of overheating, dehydration, physical exertion, stress, fatigue, or sleep deprivation. They could develop performance-degrading problems unbeknownst to their team leaders, particularly if they are fully encapsulated in chemical protective suits, are flying aircraft, or are operating in a remote location. An alert or warning signal to the individual and to his or her squad leader could permit prompt intervention to alleviate the physiological danger and potentially save a mission.
The present-day U.S. military must be able to rapidly deploy around the globe on short notice and be capable of sustaining operations for long periods of time. In order to accomplish these objectives, a variety of occupational specialties are required. Ground combat military personnel have unique roles in combat operations and work in very different environments in order to achieve objectives in a coordinated fashion. Although a thorough discussion of the challenges faced by each group of combat service members is beyond the scope of this report, the reader can gain a small appreciation for the diversity by considering the problems faced by some Army ground personnel as opposed to those faced by some pilots.
The ground personnel’s tasks often include a high level of physical energy expenditure in the face of constant and immediate threats from the environment, as well as from a wide range of enemy activities. Infantry personnel are constantly on the move, either walking, running, climbing, or at times, even swimming from one point to another. These activities are made more strenuous because of the need to carry heavy backpacks, weapons, and ammunition in all types of weather and across all types of terrain. Fatigue is a constant companion because of the physical workload, the environment, and the sleep deprivation that results from limited sleep opportunities and poor sleep environments. Air conditioning, hot water, and “normal” food are nonexistent for combat service members who often work long hours in the worst of circumstances.
The pilot’s task is generally much less physically demanding than what is faced by infantry personnel, but his or her job presents challenges from other characteristics. Helicopter pilots must remain cognitively alert at all times if they are to successfully pilot their aircraft at speeds of 50 to 100 mph while maintaining altitudes of only 50 ft above terrain obstacles. Flying close combat support means that helicopter pilots are operating under conditions that are quite similar to those faced by combat military personnel on the ground: they are close to enemy threats, they are often hot or cold due to the lack of air conditioning, and they are required to live in the most austere and uncomfortable environments for days, weeks, or months at a time. Unlike their infantry comrades, they take off and land several times a day in stressful, low-visibility conditions and try to sleep during short intervals between flights, often in poor conditions. In addition, they are responsible for multimillion-dollar aircraft and the crews on board.
Air Force fighter pilots face different, but often equally challenging circumstances. For instance, F-117 pilots have been known to fly for 18 straight hours in a single-pilot aircraft, strapped into an ejection seat while wearing bulky, hot, protective gear. During this time, they must remain fully alert and fully prepared
to engage or avoid enemy threats that arise from the air or ground while flying in a sterile, uncomfortable cockpit in a high-altitude, relatively featureless environment. Boredom and physical inactivity can decrease mental vigilance, and the fatigue from sleep deprivation and circadian disruptions are ever-present problems. Although their operational environment is generally more pleasant than the ones faced by infantry personnel, the continuous high-level cognitive demands of accomplishing the mission, combined with the responsibilities for an expensive aircraft that is fully-loaded with powerful weaponry, creates a high level of ongoing operational stress. Similar difficulties are faced by B-2 pilots who often fly missions that extend beyond 30 hours with only two pilots on board.
In addition, today’s high-performance aircraft can easily exceed the limits of human physiological tolerances. One concept for physiological monitoring includes monitoring a pilot’s approaching loss of consciousness in order to trigger an automatic take over of the plane’s controls (Forster et al., 1994). On the other hand, for flights of extended duration, detection of alertness or deterioration in performance through self and distant monitoring becomes important. This calls for a highly responsive, rapid, and reliable system that identifies any major lapse in pilot capabilities.
Navy personnel would also benefit from physiological monitoring. The Navy is designing ships that require substantially fewer crew members for operation, which in turn calls for greater reliance on each individual. Monitoring the status of these crew members becomes especially important in case they become incapacitated in an isolated compartment during high-risk damage control operations, such as fighting fires or flooding. This monitoring is part of the concept of the Reduced Ships-Crew by Virtual Presence, which is designed for smart ships that continuously receive data on the status of the ship and on the crew within the ship (Street et al., 2002).
The military would also benefit from monitoring—during both training and operations—the factors that influence bone and muscle health, as well as other processes that underlie and optimize physical endurance and resistance to physical injury. It may be most useful for leaders to use monitoring to learn the physiological and cognitive limits of each individual under their command during the individual’s training. Then, during an actual operational mission, the leaders could rely on specific warnings about real-time status. Monitoring during training may also prevent injuries. For example, if an individual is found to have reached a high state of bone and muscle remodeling during training, providing a day of rest might reduce a high probability of injury.
Physiological monitoring is also being explored for a wide variety of other military applications, including the forensic “black box” flight recorder-type of analysis (after a class A accident) of a pilot’s mental state in order to prevent future accidents (Forster, 2002). There is also a need for overall “whole-body” health markers for easy assessment of global indices of service-members’ health at regular intervals throughout their career. This could eventually include some
combination of psychological and physiological health, monitoring brain metabolites through magnetic resonance spectroscopy (MRS) scans, whole-body oxidative stress load assessments, and mitochondrial redox potential of critical brain cells as common final pathways of health status.
RECENT EVOLUTION OF MONITORING RESEARCH
Physiological monitoring concepts are not new. Fifty years ago, the Office of Naval Research and the Army Surgeon General cooperatively studied infantrymen in combat to identify metabolic predictors of mental status (Davis et al., 1952). Using neuropsychological testing (including visual flicker fusion and auditory flutter fusion tests) and blood and urine testing, hydration status, adrenal stress markers, and corresponding changes in cognitive functioning were assessed. Studies by the Air Force explored the use of an electroencephalogram (EEG) to monitor pilot performance as early as the 1950s (Sem-Jacobsen, 1959). Current studies are examining many of the same factors and relationships that were tested in the studies conducted 50 years ago.
Although the current studies have some technological advantages, most notably electronic computing power, they have largely relied on available technologies instead of exploring the most suitable measurement targets and developing specifically needed monitoring technology. To date, efforts have focused on trying to find uses for new measurement technologies instead of pushing the development of technology to systematically test the current knowledge of physiology and predict the outcomes of greatest importance.
The greatest barrier to advances in performance monitoring has been the lack of suitably defined performance outcome measures. Until recently, aviator performance has been the most extensively studied model for physiological monitoring. Military aviators have been a logical focus because of need (i.e., the high costs associated with catastrophic performance failures) and because of experimental advantages. Performance outcome measures are better defined for aviator tasks, especially the ultimate outcome of successful landing versus disaster. Also, the cockpit provides an appropriate setting for prototype monitoring systems that are power hungry and tethered to heavy equipment. Aviator studies can provide early proof of concept for systems that are later reduced in size, weight, power, and invasiveness for untethered applications in combat military personnel. Nevertheless, the aviator monitoring studies cannot be generalized without further development of performance assessment methods and metrics.
Without suitable performance measures, results from laboratory-based studies cannot be translated into militarily relevant outcomes. These measures are also needed for field studies that are otherwise forced to rely on simple dichotomies of “no bad outcome” or catastrophic failure (e.g., heat stroke, serious injury, or mission failure). The Military Operational Medicine Research Program has invested heavily in the development and standardization of practical neuropsychological tests (e.g., the Automated Neuropsychological Assessment Metric) (Kane and Kay, 1992), and current field studies are attempting to link
these test results with military performance. For example, simple reaction time remained impaired following sports concussions in military cadets even after they were cleared for return to duty by clinical criteria. The significance of this finding to other performance measures is being further investigated. Another study found that cold-water immersion affected performance tests (Vaughan, 1975); what this means to Navy diver performance capabilities is also being further investigated.
One eventual monitoring application may be to embed informative tests into common military tasks that could be monitored to obtain unobtrusive periodic assessments of an individual’s performance status. DOD is currently reviewing methods and metrics for performance assessment in order to synthesize the current state of the knowledge on militarily relevant performance assessments and models (Ness et al., In preparation). A new research initiative is focused on the development of military performance assessment methods based on measures of neurological function (e.g., voice stress analysis and eye saccades).
In 1996 physiological monitoring became a central objective in the Army research program (Friedl, 2003). The goal of the Warfighter Physiological Status Monitoring (WPSM) initiative is to make real-time performance predictions that leaders can use to assess the readiness status of their forces. The concept is to develop a combat service member-acceptable, minimally invasive sensor set with on-the-combat service member analysis. The output (which can be queried for further information) will be a simple “green” (within normal limits), “amber” (physiological challenges are present), or “red” (systems have failed and the combat service member is a casualty). This system relies on environmental physiological and psychological data that have been collected and modeled in DOD research programs for many years. A key feature of the approach is that these systems must also learn the usual range of responses for each combat service member, thus accounting for individual variability (Friedl, 2003). Currently, WPSM is a research “tool kit” to learn more about normal and abnormal physiological signals encountered in real-combat service member environments; these include a range of responses that routinely exceed those that could be obtained in an ethically developed experimental laboratory setting. The ultimate goal of WPSM will be the minimal sensor set needed for highly reliable and important predictions.
The development of experimental signal acquisition, data handling systems, and data collection studies with combat military personnel in challenging training environments is underway (Hoyt et al., 1997a, 2001). The immediate goals for WPSM are to provide information on the individual’s status for thermal strain, sleep history, energy expenditure, and live-dead detection for the Land Warrior system. More sophisticated monitoring capabilities and performance predictions are planned that will also include early casualty triage capabilities.
CURRENT RESEARCH EFFORTS
Several critical areas for metabolic monitoring have been chosen for review in this report: hydration and heat production, substrate utilization and energy metabolism, muscle and bone remodeling, and brain function. These traditionally separate research areas are interrelated through metabolic processes. For example, exertional rhabdomyolysis includes elements of hydration and heat exposure, energy flux, and muscle remodeling, with early effects on mental status (Gardner and Kark, 1994). These functions are closely interrelated through common measures that might signal changes in one or more of these physiological categories. For example, shivering may indicate a variety of threats that, when combined with one or two other measurements, can unambiguously distinguish conditions such as impending hypothermia risk, exposure to a neurotoxic chemical, or intense psychological fear. Brain function reflected in cognitive, mood, or psychomotor measures (e.g., speed of mental processing, irritability, and marksmanship) may be a common and sensitive marker of deficits of all the other stressors and functional deficits of interest, including carbohydrate metabolism in physical exhaustion (Frier, 2001), dehydration, or significant fluid shifts, such as those observed in the brain with acute mountain sickness (Singh et al., 1990), and perhaps even cytokine-mediated changes in brain function following intense muscular exertion (Febbraio and Pedersen, 2002). Thus brain function is both an early indicator of many stressors of concern and a direct reflection of specific performance capabilities.
Early metabolic changes to defend critical functions are likely to be more promising prognostic indicators than is awaiting change in the critical function itself (e.g., blood glucose, serum osmolality, or core body temperature). The critical function may be so well defended (e.g., serum osmolality and sodium concentration) that when a significant change is detected, homeostatic mechanisms have already failed and the individual is a casualty. Earlier changes in interstitial fluid or osmoregulatory hormones may signal a heroic defense against a threat to intravascular volume. There are also conditions under which a critical function measurement (e.g., body temperature) may have greater variation at performance extremes in healthy individuals, which may be appropriate compensation to sustain peak performance and defies the definitive classification of an impending performance failure. For example, core body temperature may be as low as 35ºC at the circadian nadir in U.S. Army Ranger students who have lost most of their insulative fat and have metabolically adjusted to a reduced energy intake (Hoyt et al., 1997b), and it may be sustained at 40ºC for several hours in marathoners while they are running (Maron et al., 1977). Monitoring the signs of compensation (e.g., changes in heat flux, activation of sweating or shivering mechanisms, cardiac response, and mental functioning) may predict a potential problem before unambiguous changes in core body temperature can be detected.
Bone and muscle turnover studies are important to the military to solve the near-term problem of high rates of injury during physical training—most impor-
tantly during the rapid train-up phase of the 8- to 12-week initial entry training course conducted in every service (half of all female combat service members incur musculoskeletal injury during initial entry training). A peak incidence of stress fractures by about the third week of training was hypothesized to be associated with high rates of bone remodeling stimulated by the training. This hypothesis was evaluated in an Army study that examined the benefits of a physical training rest period in the third week of training (Popovich et al., 2000). Unfortunately, the rest period did not modify the injury profile, suggesting a more complicated pathogenesis, including individual variability. The development of specific markers of susceptibility and impending injury in individuals is still urgently needed.
Table 1–1 suggests some of the outcomes that might be logical targets for monitoring within the next decade, along with some of the technologies that exist or could be developed for such monitoring. The boundary between current and near-term approaches is slightly blurred by the overlap of current technologies that require far more validation with projected near-term technologies that are just beginning to demonstrate promise. For example, fitness for duty based on various peripheral indicators of brain function is an important, but elusive, goal. In the past there was hope that performance could be predicted from recent sleep history measured by wrist-worn actigraphy (Redmond and Hegge, 1985). However, the current status of fatigue performance models is too immature and individual responses to this single measure are too variable to make actigraphy alone a useful measure. Potentially noninvasive methods that could be mounted in a helmet, such as measurement of pupil responses and saccadic eye movements, are being explored, but have so far not held up well compared with laboratory measures, such as the psychomotor vigilance task. A method that follows slow eye closure shows great promise, but will have to be proven in a helmet-type platform that keeps the monitor in line with the subject’s eyes (Dinges et al., 1998). Another potential method, voice analysis, is specifically affected by emotional load, returning to normal with psychological adaptation even while general activation (e.g., accelerated heart rate) continues (Wittels et al., 2002). However, this measure has not yet been demonstrated to correspond to specific performance decrements. EEG analyses in fatigued individuals or in individuals involved in sustained vigilance tasks have been studied in military laboratories and show promise, but remain to be demonstrated as strong predictors of impending deficits (Caldwell et al., 2002).
Far-future technologies are concepts that might be achievable but have not been seriously explored and remain “marks on the wall.” Mitochondrial redox state in specific brain tissues has been suggested a marker of brain function status based on the importance of neural cell bioenergetics (Ojaimi et al., 1999). Intracerebral monitoring of energy-related metabolites is currently being conducted with neurosurgical patients to follow acute conditions involving hypoxia and ischemia. As more is learned about what needs to be measured, the
TABLE 1–1 Technology Forecast for Practical Metabolic Assessment Measures (Measured Endpoints and Conceivable Technologies)
Past |
Presenta |
Energy balance and fuel availability |
|
Blood and urine biochemistry Home test glucose monitors, laboratory tests Ratings of perceived exertion |
Activity-based predictions “Gluco-watch” Reverse iontophoresis, actigraphy |
Brain metabolic function |
|
Paper and pencil tests |
Computerized neuropsychological testing EEG spectral analysis Palm-top test, dry electrodes in a hat band |
Hydration and water balance |
|
Urine-specific gravity |
Balance based on intake and predicted losses Instrumented canteen/camelbak, bioelectrical resistance Whole-body water estimates |
Bone and muscle turnover |
|
“Hot spots” Loss of strength and delayed onset muscle soreness Thermography |
Lab tests Specific blood and urinary markers (e.g., telopeptides, myoglobin, CPK, IGF-1) |
Stress and Immune Function |
|
Psychological measures of: performance, stress, mood Sleep patterns Stress hormones |
Blood, urine, and saliva immune markers (e.g., ILs, NK cells, T-cells, IGs, neutrophils, macrophages) Blood, urine, and saliva stress markers (cortisol, ACTH, catecholamines) Heart rate variability |
aEEG=electroencephalogram, CPK=creatine phosphokinase, IGF-1=insulin-like growth factor-1, IL=interleukin, NK=natural killer, IG=immunoglobulin, ACTH= adrenocorticotropic hormone. |
technologists may be able to develop the noninvasive monitoring devices needed to monitor the identified biomarkers. For example, with higher-powered magnets, researchers are now able to detect glutamate peaks in MRS brain pixels. An elevated level of glutamate in the frontal lobe might signify a range of acute metabolic insults that would be very important to detect and countermand.
Current military research programs are leveraged with special Congressional appropriations that accelerate basic metabolic research in specific topic areas. The Bone Health and Military Medical Readiness research program (supported by the National Osteoporosis and Related Bone Disorders Coalition) is
Near Futureb |
Far Futurec |
Semi-invasive implantable sensors and “tattoos” Subdermal continuous glucose, lactate, pH, free fatty acids |
Functional outcome (e.g., EMG, nerve conduction, changes in thermal flux) Noninvasive physiological sensors built into clothing |
Saccades and pupil responses Voice analysis Task-embedded psychological tests Doppler, etc., in combat service member helmet/spectacles |
Brain blood flow Chemical nose, respiratory sampling, personal intrahelmet brain imaging systems Sweat/exhaled cytokines Volatile compounds/pheromones |
Intercellular fluid assessment Subdermal wicks, boot-sensor body weight tracking with electrolyte and BIA sensors Whole body water changes |
Changes in skin properties Endocrine changes in defense of water volume Skin mechanical/electrical changes, semiinvasive sensing of osmoregulatory hormones |
Altered biomechanics Sweat markers of calcium and protein metabolism Practical field test systems |
Changes in redox status Deep muscle biochemical sensors Regional metabolism/blood flow changes |
Field measures of: Blood, urine, or saliva immune markers (e.g., ILs, IGs) Blood, urine, or saliva stress markers (cortisol, ACTH, catecholamines) Sleep patterns |
Human odors Sweat immune and stress biomarkers (e.g., neuropeptides, neurohormones, ILs, IGs) |
b BIA=bioelectrical impedance analysis. c EMG=electromyogram. SOURCE: Friedl (2003). |
focused on the improved understanding of bone remodeling processes and includes projects exploring markers of impending stress fracture injury. The Technologies for Metabolic Monitoring research program (supported by the Juvenile Diabetes Research Foundation) is testing novel approaches to measure functional outcomes related to biochemical status and energy metabolism, notably glucose regulation, but including the development of lactate sensors and the exploration of other physiological indicators of metabolic status. Projects supported by the Force Health Protection research program are examining methods to monitor global health status in combat service members, including the use of breath condensates to measure cytokines and other markers of lung function
following blast or toxic inhalation exposures. Two large projects are currently in progress to assess the association of brain MRS measures and symptom reporting in chronic, multisymptom illnesses in order to determine objective markers of well-being. Finally, the Neurotoxin Exposure Treatment Research Program (sponsored by the Parkinson’s Action Network) includes the exploration of voice analysis and neuropsychological testing methods for the early detection of neurological changes.
CURRENT STATUS OF FIELD APPLICATIONS OF PHYSIOLOGICAL MONITORING
The dismounted combat service member’s workplace is fairly unique within the variety of occupational challenges encountered by the American population. Modern infantry combat service members commonly engage in intense, mentally and physically demanding, 3- to 10-day missions, often in rugged terrain or complex urban settings. These individuals carry heavy loads (35–65 kg) and are often food- and sleep-restricted. Environmental conditions—ambient temperature, humidity, wind speed, solar load, and barometric pressure—can vary widely. Recent examples of operational environments include the desert heat conditions of the Persian Gulf; cold, wet weather in Bosnia; and cold and high altitude challenges in the mountains of Afghanistan.
The WPSM concept includes wearable metabolic and physiological status monitors to help improve combat service members’ performance. This monitoring plays important roles in: (a) sustaining physical and mental performance; (b) reducing the likelihood of nonbattle injuries, such as heat stroke, frostbite, and acute mountain sickness; and (c) improving casualty management in remote situations.
Ambulatory WPSM technologies are being developed to provide useful performance and health status indicators for combat service members, medics, commanders, and logisticians. The WPSM program uses a novel research “tool kit” to collect ambulatory physiological data from military personnel operating in stressful field environments. Analyses of the data sets are providing a better understanding of the physiological strains associated with operations in a multistressor environment.
Sensor hardware is important in ambulatory metabolic and physiological monitoring. However, sensor development is only one of a series of steps needed to reliably generate a useful flow of health status information in a harsh and highly constrained wearable environment. Other steps include: reliable sensor data collection, data cleaning, data reduction and interpretation, and the communication, synthesis, interpretation, and presentation of the data. Key technologies that support this process, including post-hoc, time-series data management and the medical Personal Area Network, are reviewed elsewhere (Hoyt et al., 2002).
Power, weight, and volume constraints and the need for truly “wear-and-forget” comfort limit the functionality of wearable sensors. For example, esti-
mating sleep by monitoring activity is possible, but it is not currently practical to do so by EEG in the field. An intelligent sensor network that reliably generates useful information from a number of disparate sources is needed to provide a holistic, rather than a “keyhole,” view of the physiological status of individuals.
THE PHYSIOLOGICAL STATUS MONITOR
A prototype WPSM user interface (display) for the medic or field commander (Figure 1–1) illustrates the relevant types of contextual and physiological information that are available. This heuristic display shows: (1) thermal/work strain as the Physiological Strain Index (PSI) (Moran et al, 1998), (2) hydration state or water balance, (3) metabolic rate, (4) environmental conditions, (5) cognitive/sleep status (hours of sleep, etc.), and (6) clinical status and location information. The knowledge display includes a baseline characterization of the individual, real-time combat service member and environmental sensor input, and historical and group mean data. Some of the measurements provided by the WPSM and their potential uses are described in the following sections.
Combat Service Member Characteristics
Combat service member characteristics, along with clothing, diet, load, geolocation, and meteorological conditions (air temperature, solar load, wind speed, and humidity), are important determinants of an individual’s physiological and pathophysiological responses to environmental stresses and trauma. Relevant combat service member characteristics include job type (military occupational specialty), gender, ethnicity, age, height, body weight, percent body fat, thermal and altitude acclimation history, and aerobic fitness. These factors change slowly, if at all, and can be recorded well before any training or combat mission begins. Percent body fat can be estimated easily from weight and waist circumference (Wright and Wilmore, 1974), but simple field techniques for characterizing thermal and altitude acclimation states are currently not well defined. Aerobic fitness can be estimated from the Army Physical Fitness Test 2-mile-run-for-time score (Mello et al., 1988) or from foot-ground contact time and heart rate using the method of Weyand and colleagues (2001).
Heat Strain
Understanding why hot weather injuries occur and developing ways to prevent heat injuries are important concerns given the approximately 1,800 heat injuries that occurred in active-duty combat service members in the Army in 2002 (USACHPPM, 2003). The graphic display in Figure 1–2 shows core temperature, measured by an ingested radio telemetry pill (O’Brien et al., 1998), and heart rate, typically derived from an electrocardiogram. The PSI (lumped core temperature) and heart rate index that reflects thermal and work strain on a scale
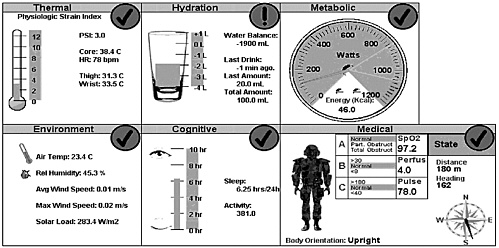
FIGURE 1–1 Prototype combat service member physiological status monitoring user interface (display) for the medic or field commander illustrating contextual and physiological information. This heuristic display shows (1) thermal/work strain as physiological strain index (PSI), (2) hydration state or water balance, (3) metabolioc rate, (4) enviromental conditions, (5) cognitive/sleep status (hours of sleep, etc.), and (6) clinical status and location information.
SOURCE: Hoyt and Friedl (2003).
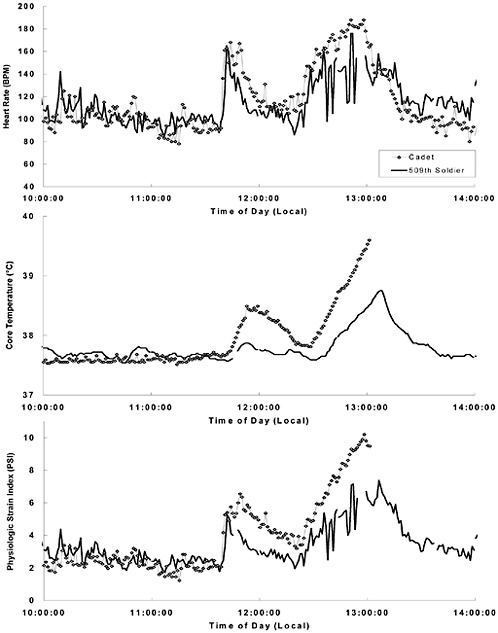
FIGURE 1–2 Heart rate, core temperature, and physiological strain index in two combat service members engaged in similar training activities during a hot-weather field exercise at the Joint Readiness Training Center, Ft. Polk, Louisiana. The thermal/work strain levels associated with two bouts of marching (1145–1200 h and 1230–1300 h) were more pronounced in the heat-exhaustion casualty (cadet) than in the less-affected 509th combat service member. The heat casualty had a higher body fat percent, carried a heavier load, was less physically fit, and was not heat acclimated as compared with his 509th cohort.
SOURCE: Hoyt and Friedl (2003).
of 0 to 10 (Moran et al., 1998) is currently used to generate green, amber, or red alerts as thresholds are passed. PSI values may prove useful in assessing acclimation status, guiding heat acclimation routines, and setting the timing and duration of work/rest cycles. A prototype thermal strain model, SCENARIO, estimates core temperature from work rate, clothing characteristics, and ambient meteorological conditions (Kraning and Gonzalez, 1997). This and other surrogate measures of core temperature may be appropriate when risk of hypo- or hyperthermia is moderate and more precise core temperature measurements, such as those provided by the ingested radio telemetry pill, are not needed. The core temperature requirement is likely to be replaced by improvements in heat flux modeling from measures of cutaneous responses and temperatures. Combined with other sensor measurements, cutaneous responses and temperature may provide strong inferences not only about thermal status, but also about shock and hemorrhage.
Cold Strain
Cold injuries, that is, hypothermia and peripheral cold injuries are also a major concern for combat service members (USACHPPM, 2001). Temperature radio telemetry pills can be used to monitor for hypothermia (O’Brien et al., 1998). Peripheral temperature and heat flux sensors can be used to assess the risk of peripheral cold injury and to guide improvements in clothing, boots, and gloves. The Cold Strain Index (Moran et al., 1999) uses core and peripheral temperatures to track cold strain. However, this algorithm needs to be modified to account for altered thermoregulation during underfeeding and sleep. (For a discussion of physiological adjustment of humans to the cold, see Toner and McArdle, 1988.)
Hydration
Under- or overhydration can lead to decrements in physical and cognitive performance, increased risk of heat injury, hyponatremia, and death (Montain et al., 2001; Sawka, 1988). Mission water requirements, largely driven by basal water needs and sweat losses, can be predicted based on the anticipated weather, clothing, load weight, and metabolic rate during the mission (Kraning and Gonzalez, 1997). Technologies to monitor water intake from bladder-type canteens, the “drink-o-meter” concept, can help ensure adequate water intake (water discipline). However, practical field methods to assess overall body tissue hydration or to monitor hydration through urine output have yet to be developed. Tests of the use of body resistance measurements have consistently failed to demonstrate accurate tracking of water changes, perhaps in part because of the inability to control for variability in electrolyte concentrations during various
types of dehydration (Berneis and Keller, 2000; Koulmann et al., 2000). It may be possible in the future to improve electrical resistance-derived estimates of hydration with minimally invasive subdermal electrolyte sensors. Alternatively, future automatic monitoring of urinary excretion rates and solute concentrations may provide valuable insight into hydration status and other aspects of acute combat service member health.
Metabolic Status and Energy Reserve: Modeling Metabolic Fuel Requirements
Field rations may not always meet the nutritional needs of combat service members (Friedl and Hoyt, 1997). Negative energy balance, commonly associated with undereating in the field, can usually be managed with little consequence by drawing on substantial body fat reserves. Body energy reserves can be calculated from percent body fat (estimated from waist circumference) less the 5 percent absolute minimum body fat levels attainable in underfed, healthy male combat service members (Friedl et al., 1994). However, negative carbohydrate balance, which is common in the field and is associated with decreased endurance capacity and loss of lean mass, is more difficult to manage due to the body’s limited carbohydrate reserves.
Carbohydrate requirements can be estimated from aerobic fitness, daily activity patterns, and the metabolic cost of locomotion (Hoyt and Weyand, 1997). Maximum aerobic capacity can be derived from the Army’s Annual Physical Fitness Test 2-mile-run-for-time results (Mello et al., 1988), and daily activity patterns can be derived from heart rate or actigraphy (Redmond and Hegge, 1985). The metabolic cost of locomotion can be derived from total weight and foot-ground contact times (pedometry) (Hoyt and Weyand, 1997; Kram and Taylor, 1990) or from the Pandolf equation, which includes body weight, load weight, and geolocation (including velocity of movement, grade, and footing) (Pandolf et al., 1977). Knowing metabolic rate and the maximum aerobic capacity for each individual, an exercise intensity profile can be generated (i.e., percent of maximum aerobic capacity over time). Oxygen consumption can be partitioned into carbohydrate and fat combustion by assuming a given relationship between resting or exercise intensity and nonprotein respiratory exchange ratio (carbon dioxide production/oxygen consumption) and using standard conversion factors. The exercise intensity-respiratory exchange ratio relationship chosen might be more fat-predominant than that of fully fed individuals (Åstrand and Rodahl, 1986) due to practical limits on the amount of food that can be carried.
Remote Trauma Triage
Combat service members are expected to be widely dispersed on the battlefield and often minimal medical care is available to combat casualties. To help improve remote casualty management, a remote trauma triage system is being
developed. This system, part of the WPSM, will contain sensors and algorithms that allow medics to remotely detect ballistic wounding events and to determine casualty life signs and the need for a major surgical life-saving intervention (Holcomb et al., In press). Parameters important in life-sign detection after wounding include responsiveness to radio contact, motion, body position, cardiac activity, and systolic blood pressure. Distilled health status information will foster the effective use of medical resources (e.g., time, equipment, and supplies).
Altitude Acclimatization
Combat service members deploying to elevations above 2,800 m (~ 8,000 ft) may experience Acute Mountain Sickness (Pandolf et al., 1988), which is characterized by headache, nausea, fatigue, decreased appetite, and poor sleep, often with signs of poor balance and mild swelling of the face, hands, and feet. Without special preparation, a large proportion of a military unit rapidly inserted at high altitude is likely to develop acutely debilitating symptoms. Normally, Acute Mountain Sickness is either absent or resolves within 3 to 7 days following ascent. However, maladaptation can lead to life-threatening high-altitude pulmonary or cerebral edema. An individual’s acclimatization state can be assessed by comparing blood-oxygen saturation for a given ascent profile (i.e., SaO2 for the reported or measured exposure to hypobaric hypoxia) with that expected in normal acclimatization. An ability to monitor and model acclimatization status will make it easier to plan high-altitude missions and minimize altitude illnesses.
EXAMPLE APPLICATION: CHARACTERISTICS OF A HEAT CASUALTY
Heat strain provides a demonstration of nascent capabilities for physiological monitoring. Progressive heat strain moves on a continuum from impaired cognitive function to actual casualty status and presents one of the first opportunities to provide commanders with useful predictions of failing performance before a combat service member becomes an environmental stress casualty. Collection of field data that includes clear medical outcomes makes it possible to backtrack to earlier indicators of the impending health risk and to develop more precise predictive thresholds of individual risk.
Hoyt and Friedl (2003) discussed a recent example that studied individual responses to heat stress. A pair of combat service members were engaged in similar training activities during a hot-weather field exercise at the Joint Readiness Training Center, Ft. Polk, Louisiana. Although the two combat service members performed similar activities from about 1130 to 1400 h (ambient temperature=32º–34ºC; relative humidity=46–55 percent; solar load=800–875
TABLE 1–2 Age, Physical Characteristics, Total Load Carried, and Maximal Aerobic Capacity of Two Combat Service Members—A Heat Exhaustion Casualty (Cadet) and an Unaffected 509th Combat Service Member from the 1/509th Infantry Brigade (Airborne)
W/m2; wind speed=1–2 ms−1), and both were fed and hydrated, only one became a heat casualty. Combat service member characteristics (Table 1–2), including analysis showed that the combat service members’ differences in response to the heat stress were due to a number of factors. The heat casualty had a higher percent body fat, carried a heavier load, was less physically fit, and was not heat acclimated (by interview) as compared with his unaffected cohort. These results illustrate the importance of integrating multiple data streams in the process of understanding multistressor physiological events.
As illustrated in the paragraph above, individuals respond differently to similar work loads in hot environments. In the example, the differences in body size, level of fitness, and acclimation to the heat appeared to be the primary factors contributing to one combat service member being overcome by the heat while the other was fine. Based on the extensive research literature, it is known that a number of factors determine how a particular individual responds to exercise in a hot environment. These factors include the environment (temperature, altitude, wind chill index, humidity), physical factors associated with the individual (body temperature, level of acclimation, fluid intake, level of fitness, level of exercise intensity required to do the work), and the individual’s overall health and well-being. Those who are well fed and hydrated, are fit and adapted to the environment, and are wearing appropriate clothing will typically fair better than those who are not. Understanding how individuals respond to specific environmental challenges will aid in training individuals to monitor their own well-being and make appropriate change so that heat stress does not occur.
REFERENCES
Åstrand PO, Rodahl K. 1986. Textbook of Work Physiology. Physiological Bases of Exercise. 3rd ed. New York: McGraw-Hill Book Co.
Berneis K, Keller U. 2000. Bioelectrical impedance analysis during acute changes of extracellular osmolality in man. Clin Nutr 19:361–366.
Caldwell JA, Hall KK, Erickson BS. 2002. EEG data collected from helicopter pilots in flight are sufficiently sensitive to detect increased fatigue from sleep deprivation. Int J Aviat Psychol 12:19–32.
Davis SW, Elmadjian F, Hanson LF, Liddell HS, Zilinsky AA, Johnston ME, Killbuck JH, Pace N, Schaffer FL, Walker EL, Minard D, Kolovos ER, Longley GH. 1952. A Study of Combat Stress, Korea 1952. Technical Memorandum ORO-T-41(FEC). Chevy Chase, MD: Operations Research Office, The Johns Hopkins University.
Dinges DF, Mallis MM, Maislin G, Powell JW. 1998. Evaluation of Techniques for Ocular Measurement as an Index of Fatigue and as the Basis for Alertness Management. DOT-HS-808–762. Washington, DC: National Highway Traffic Safety Administration.
DOD (U.S. Department of Defense). 2001. Annual Report to the President and the Congress. Washington, DC: U.S. Government Printing Office.
Febbraio MA, Pedersen BK. 2002. Muscle-derived interleukin-6: Mechanisms for activation and possible biological roles. FASEB J 16:1335–1347.
Forster EM. 2002. Safety of Flight: The Physiologic Aspect of the Weapon System. Patuxent River, MD: Naval Air Warfare Center Aircraft Division.
Forster EM, Morrison JG, Hitchcock EM, Scerbo MW. 1994. Physiologic Instrumentation in the Naval Air Warfare Center Human-Use Centrifuge to Determine the Effects of Cumulative+Gz on Cognitive Performance. NAWCADWAR-95006–4-6. Warminster, PA: Naval Air Warfare Center Aircraft Division.
Friedl KE. 2003. Predicting and Protecting Performance using Metabolic Monitoring Strategies: It’s All Wet Stuff Anyway, Isn’t It? Presented at the Institute of Medicine, Committee on Metabolic Monitoring Technologies for Military Field Applications Workshop on Metabolic Monitoring Technologies for Military Field Applications, San Antonio, Texas, January 8–9.
Friedl KE, Hoyt RW. 1997. Development and biomedical testing of military operational rations. Annu Rev Nutr 17:51–75.
Friedl KE, Moore RJ, Martinez-Lopez LE, Vogel JA, Askew EW, Marchitelli LJ, Hoyt RW, Gordon CC. 1994. Lower limit of body fat in healthy active men. J Appl Physiol 77:933–940.
Frier BM. 2001. Hypoglycaemia and cognitive function in diabetes. Int J Clin Pract 123:30–37.
Gardner JW, Kark JA. 1994. Fatal rhabdomyolysis presenting as mild heat illness in military training. Mil Med 159:160–163.
Holcomb JB, Niles SE, Hinds D, Aoki N, Salinas J, Flannigan TJ, Macaitis JM, Duke JH, Moore FA. In press. Prehospital physiologic data and life saving interventions in trauma patients. J Trauma.
Hoyt RW, Friedl KE. 2003. Current Status of Field Applications of Physiological Monitoring for the Dismounted Soldier. Presented at the Institute of Medicine, Committee on Metabolic Monitoring Technologies for Military Field Applications Workshop on Metabolic Monitoring Technologies for Military Field Applications, San Antonio, Texas, January 8–9.
Hoyt RW, Weyand PG. 1997. Advances in ambulatory monitoring: Using foot contact time to estimate the metabolic cost of locomotion. In: Carlson-Newberry SJ, Costello RB, eds. Emerging Technologies for Nutrition Research. Washington, DC: National Academy Press. Pp. 315–343.
Hoyt RW, Buller M, Redin MS, Poor RD, Oliver SR. 1997a. Soldier Physiological Monitoring—Results of Dismounted Battlespace Battle Lab Concept Experimentation Program Field Study. Natick, MA: U.S. Army Research Institute of Environmental Medicine.
Hoyt RW, Young AJ, Matthew WT, Kain JE, Buller M. 1997b. Warfighter Physiological Status Monitoring (WPSM): Body Core Temperatures during 96 H of Swamp Phase Ranger Training. Technical Report T97–4. Natick, MA: U.S. Army Research Institute of Environmental Medicine.
Hoyt RW, Buller MJ, DeLany JP, Stultz D, Warren K. 2001. Warfighter Physiological Status Monitoring (WPSM): Energy Balance and Thermal Status during a 10-day Cold Weather U.S. Marine Corps Infantry Officer Course Field Exercise. Natick, MA: U.S. Army Research Institute of Environmental Medicine.
Hoyt RW, Reifman J, Coster TS, Buller MJ. 2002. Combat medical informatics: Present and future. In: Kohane IS, ed. Biomedical Informatics: One Discipline. Proceedings of the 2002 AMIA Annual Symposium, November 9–13, 2002, San Antonio, Texas. Bethesda, MD: American Medical Informatics Association. Pp. 2335–2339.
Kane RL, Kay GG. 1992. Computerized assessment in neuropsychology: A review of tests and test batteries. Neuropsychol Rev 3:1–117.
Koulmann N, Jimenez C, Regal D, Bolliet P, Launay JC, Savourey G, Melin B. 2000. Use of bioelectrical impedance analysis to estimate body fluid compartments after acute variations of the body hydration level. Med Sci Sports Exerc 32:857–864.
Kram R, Taylor CR. 1990. Energetics of running: A new perspective. Nature 346:265–267.
Kraning KK, Gonzalez RR. 1997. A mechanistic computer simulation of human work in heat that accounts for physical and physiological effects of clothing, aerobic fitness, and progressive dehydration. J Therm Biol 22:331–342.
Maron MB, Wagner JA, Horvath SM. 1977. Thermoregulatory responses during competitive marathon running. J Appl Physiol 42:909–914.
Mello RP, Murphy MM, Vogel JA. 1988. Relationship between a two mile run for time and maximal oxygen uptake. J Appl Sport Sci Res 2:9–12.
Montain SJ, Sawka MN, Wenger CB. 2001. Hyponatremia associated with exercise: Risk factors and pathogenesis. Exerc Sports Sci Rev 29:113–117.
Moran DS, Shitzer A, Pandolf KB. 1998. A physiological strain index to evaluate heat stress. Am J Physiol 275:R129–R134.
Moran DS, Castellani JW, O’Brien C, Young AJ, Pandolf KB. 1999. Evaluating physiological strain during cold exposure using a new cold strain index. Am J Physiol 277:R556–R564.
O’Brien C, Hoyt RW, Buller MJ, Castellani JW, Young AJ. 1998. Telemetry pill measurement of core temperature in humans during active heating and cooling. Med Sci Sports Exerc 30:468–472.
Ojaimi J, Masters CL, Opeskin K, McKelvie P, Byrne E. 1999. Mitochondrial respiratory chain activity in the human brain as a function of age. Mech Ageing Dev 111:39–47.
Pandolf KB, Givoni B, Goldman RF. 1977. Predicting energy expenditure with loads while standing or walking very slowly. J Appl Physiol 43:577–581.
Pandolf KB, Sawka MN, Gonzalez RR, eds. 1988. Human Performance Physiology and Environmental Medicine at Terrestrial Extremes. Indianapolis, IN: Benchmark Press.
Popovich RM, Gardner JW, Potter R, Knapik JJ, Jones BH. 2000. Effect of rest from running on overuse injuries in army basic training. Am J Prev Med 18:147–155.
Redmond DP, Hegge FW. 1985. Observations on the design and specification of a wrist-worn human activity monitoring system. Behav Res Methods Instrum Comp 17:659–669.
Sawka MN. 1988. Body fluid responses and hypohydration during exercise-heat stress. In: Pandolf KB, Sawka MN, Gonzalez RR, eds. Human Performance Physiology and Environmental Medicine at Terrestrial Extremes. Indianapolis, IN: Benchmark Press. Pp. 227–266.
Sem-Jacobsen CW. 1959. Electroencephalographic study of pilot stresses in flight. J Aviat Med 30:797–801.
Singh MV, Rawal SB, Tyagi AK. 1990. Body fluid status on induction, reinduction and prolonged stay at high altitude of human volunteers. Int J Biometerol 34:93–97.
Street TT, Nguyen X, Williams FW. 2002. Wireless Communication Technologies on Ex-USS Shadwell NRL-MR-6180–02–8631. Washington, DC: Naval Research Laboratory.
Toner MM, McArdle WD. 1988. Physiological adjustments of man to the cold. In: Pandolf KB, Sawka MN, Gonzalez RR, eds. Human Performance Physiology and Environmental Medicine at Terrestrial Extremes. Indianapolis, IN: Benchmark Press. Pp. 361–399.
USACHPPM (U.S. Army Center for Health Promotion and Preventive Medicine). 2001. Cold weather injuries among active duty soldiers, U.S. Army, 1997–2001. Med Surveill Month Rep 7:2–5.
USACHPPM. 2003. Heat-related injuries, U.S. Army, 2002. Med Surveill Month Rep 9:2–4.
Vaughan WS Jr. 1975. Diver temperature and performance changes during long-duration, cold water exposure. Undersea Biomed Res 2:75–88
Weyand PG, Kelly M, Blackadar T, Darley JC, Oliver SR, Ohlenbusch NE, Joffe SW, Hoyt RW. 2001. Ambulatory estimates of maximal aerobic power from foot-ground contact times and heart rates in running humans. J Appl Physiol 91:451–458.
Wittels P, Johannes B, Enne R, Kirsch K, Gunga HC. 2002. Voice monitoring to measure emotional load during short-term stress. Eur J Appl Physiol 87:278–282.
Wright HF, Wilmore JH. 1974. Estimation of relative body fat and lean body weight in a United States Marine Corps population. Aerospace Med 45:301–306.