Executive Summary
At hundreds of thousands of commercial, industrial, and military sites across the country, subsurface materials including groundwater are contaminated with chemical wastes. Although many hazardous waste sites have been cleaned up since enactment of the Superfund regulations in the 1980s, many sites with recalcitrant organic contaminants remain in exceedance of water quality and soil standards. For a number of reasons, these sites have proven resistant to early remediation efforts such as pump-and-treat technology. Subsurface heterogeneities, contaminant sorption onto aquifer solids, and contaminant diffusion into low-permeability zones combine to make pump-and-treat much less efficient than originally envisioned. Furthermore, many organic pollutants have low water solubility and tend to remain in the subsurface as either a separate organic phase liquid (nonaqueous phase liquid or NAPL) or separate solid phase. Where they are present, separate phase or sorbed contaminants serve as a long-lived contamination source to groundwater.
The technical difficulties involved in characterizing and remediating source zones and the potential costs are so significant that there have been no reported cases of large DNAPL (dense nonaqueous phase liquid) sites where remediation has restored the site to drinking water standards. Nonetheless, pressure from the affected public to clean up these sites and a desire on the part of responsible parties to reach site closure remain, such that in the last few years, certain technologies capable of significant source remediation are being increasingly utilized by large responsible parties, like the U.S. military. In particular, the Army Environmental Center, which coordinates the Army’s efforts to restore thousands of contaminated sites at installations across the country, requested the National
Research Council’s (NRC) input to help determine the usefulness and applicability of source remediation as a cleanup strategy, including what can be accomplished by more aggressive technologies in terms of the total contaminant mass removed, risk reduction, and other metrics. Although chlorinated solvent DNAPLs are the primary focus of the report, chemical explosives are also considered in depth. The statement of task is provided below:
-
What is a meaningful definition of a “source” for the purpose of this study? How important is the source delineation step to the effectiveness of mass removal as a cleanup strategy? What tools or methods are available to delineate sources of organics contamination in complex sites? How should the uncertainty of these characterizations be quantified, in terms of both total mass and mass distribution?
-
What are the data and analytical requirements for determining the effectiveness of various source removal strategies, and how do these requirements change for different organic contaminant types or hydrogeologic environments? Effectiveness would consider the metrics of groundwater restoration, plume shrinkage and containment, mass removed, risk reduction, and life cycle site management costs.
-
What tools or techniques exist today, and what tools would need to be developed in the future, to help predict the likely benefits of source removal?
-
What would be the most important elements of a well-designed protocol to assist project managers in the field to assess the effects of source removal?
-
What can be concluded about the ability of source removal efforts to bring about substantial water quality benefits and to meet various cleanup goals? (For example, when can these efforts remove enough of the source to then rely on monitored natural attenuation?)
-
What have been the results of source removal activities at Army and other facilities to date? More generally, what can be said about the future use of source removal as a cleanup strategy and the specific technologies investigated during the study?
SOURCE ZONES
As a preliminary step, the NRC committee formed to conduct the study created a definition of “source” that would capture the essence of a source as a reservoir of contamination while making a distinction between the source zone and the plume of contaminated groundwater. In addition, to better capture the properties of chemical explosives, the definition encompasses pure solid sources, as shown below:
A source zone is a saturated or unsaturated subsurface zone containing hazardous substances, pollutants, or contaminants that acts as a reservoir
that sustains a contaminant plume in groundwater, surface water, or air, or acts as a source for direct exposure. This volume is or has been in contact with separate phase contaminant (NAPL or solid). Source zone mass can include sorbed and aqueous phase contaminants as well as contamination that exists as a solid or NAPL.
Understanding the characteristics of subsurface source zones is critical to effectively conducting both site characterization and remediation. The nature of the hydrogeologic environment, the composition and release of the chemical contaminants, and subsequent transport and transformation processes in the subsurface combine to determine how contaminants are distributed within source zones. Five hydrogeologic settings, described in Chapter 2, are broadly representative of the common conditions of concern:
-
Type I granular media with low heterogeneity and moderate to high permeability
-
Type II granular media with low heterogeneity and low permeability
-
Type III granular media with moderate to high heterogeneity
-
Type IV fractured media with low matrix porosity
-
Type V fractured media with high matrix porosity
These settings differ in their permeability, heterogeneity, and porosity—parameters that control how contaminants are stored and released from source zones under natural and engineered conditions. For example, fractured media sites characterized by high matrix porosity (Type V) tend to store contaminants in stagnant aqueous zones and sorbed to aquifer solids. Reverse diffusion of contaminants from these areas can sustain elevated contaminant concentrations in groundwater for long periods of time. The scale of the representative hydrogeologic settings is in the range of a few meters, whereas the size of an entire source zone can be on the order of tens of meters. Source zones can occur within a single hydrogeologic setting (e.g., a sand dune deposit) or can include multiple hydrogeologic settings (e.g., alluvium overlying fractured crystalline rock). In addition to determining the overall subsurface distribution of contamination, the existing hydrogeologic setting limits both the types of tools that can be used to characterize the source zone and the technologies that might achieve reductions in source mass.
Organic contaminants are typically released to the subsurface as constituents of a liquid phase, such as a dilute aqueous solution, a concentrated aqueous solution (leachate), or an organic liquid (NAPL) that is immiscible with water. In the case of chlorinated solvents that form DNAPLs, migration in the subsurface is controlled by the liquid’s density and viscosity and its interfacial tension with the pore water, as well as by properties of the formation solids, including texture and wettability. DNAPLs can form pools in the subsurface, or they can exist as small
globules or ganglia retained within the aquifer pores. Among the many distinguishing features of DNAPL sites is the fact that the distribution of DNAPL in the subsurface is typically sparse and highly heterogeneous. Once contaminants are in the subsurface, processes such as dissolution, sorption, and biodegradation work to further affect contaminant distribution by redistributing mass locally among phases as well as carrying the contaminant away from the site of initial release. Depending on the hydrogeologic setting, a portion of the contaminant mass released to the subsurface as a DNAPL may diffuse into stagnant zones as either sorbed or dissolved phase contamination.
Compared to DNAPLs, the characteristics of chemical explosive source zones are less well understood, partly because of the safety issues involved in characterizing these sites. Nonetheless, it is thought that most chemical explosives from production and manufacturing process discharges are released to the environment as aqueous mixtures, from which the compounds precipitate out, usually within 6 m (20 ft) of the soil surface. Some highly concentrated wastes in production process discharges might act like DNAPLs or dense miscible phase liquids. However, even these explosive materials are likely to undergo significant change once they are introduced into soil, where environmental conditions would tend to decrease both temperature and acidity, promoting the creation of a separate solid phase material. Recharge of the subsurface during rain events can lead to dissolution of solid phase explosives and subsequent transfer of explosives mass to soil pore water and perhaps groundwater.
For both chlorinated solvent and explosives source zones, contaminant plumes develop downgradient of the source material in cases where the contaminants are soluble in water and are resistant to natural biodegradation. In general, groundwater plumes tend to have larger spatial extents and to be more continuous in nature in comparison to contaminant mass distributions within source zones. Over time, biogeochemical processes in the plume can result in a contaminant mixture with a very different composition than the original release materials. It should be noted that sorption or diffusion of contaminants from the plume onto aquifer solids (and subsequent reverse diffusion) is common in many hydrogeologic settings. Although this sorbed or stagnant-zone mass can be a chronic supply of aqueous phase contamination, it does not constitute a source zone (as herein defined) because it does not exist where the DNAPL or solid phase was present. Therefore, not all groundwater contaminant plumes imply the presence of a source zone.
SOURCE ZONE CHARACTERIZATION
The hydrogeologic environment and contaminant distribution of a hazardous waste site are revealed through site characterization—a continuous, dynamic process of building and revising a site conceptual model that captures all aspects of the site, including the source zone. Chapter 3 addresses several aspects of
source zone characterization, including the potential ramifications of inadequate characterization, characterization methods and tools, the importance of source zone characterization to determining cleanup objectives, scale issues, and coping with uncertainties during source characterization. A recurring theme is that source zone characterization should be carried out in a manner that best informs the entire source remediation process. Decisions regarding the objectives of remediation and the remediation technologies selected will have a strong impact on the source zone characterization strategy and vice versa.
Although it is impossible to prescribe a specific step-by-step source zone characterization process because of differing conditions from site to site, there are four broad categories of information that are critical to characterizing all source zones:
-
Understanding source presence and nature. What are the components of the source, whether a DNAPL or explosive material, and what is the expected behavior of the individual components based on known information?
-
Characterizing hydrogeology. What are the lithology of the subsurface and groundwater flow characteristics as they pertain to the source zone? Are there multiple aquifers at the site, and how are they connected? What are the properties and connectedness of the low-permeability layers or zones? Can the flow system be described at the specific site and at a larger scale? Can the groundwater velocity and direction (and the spatial and temporal variation in both) be measured?
-
Determining source zone geometry, distribution, migration, and dissolution rate. Where is the source with respect to lithology? Is it present as pooled DNAPL, distributed as residual saturation, or both? Is it crystalline explosive material or is it sorbed? What is the current vertical and lateral extent of the source material, and what is the potential for future migration based on the hydrogeologic characteristics of the site? How fast is the source dissolving?
-
Understanding the biogeochemistry. What roles do transport and transformation processes play in attenuating the source zone and the downgradient plume? How will possible remediation strategies affect the geochemical environment (e.g., by releasing other toxic substances, or by adding or removing substances upon which microbial activity and contaminant degradation depend)?
Although there may be an overall work plan directing that source characterization activities be conducted in a particular order, each of the activities is related to the others, and a good deal of iteration between the general categories is not only desirable but critical to the process.
For each of these four primary categories of information, Chapter 3 outlines characterization methods and tools that can be used, including noninvasive characterization approaches (ranging from collecting historical information to certain geophysical techniques), invasive sampling tools, methods for laboratory analysis, and tools that represent a combination of the above. Some of the tools are
approaches to removing contaminant samples from the subsurface, some measure specific chemicals either in situ or following sample extraction, some perform both functions, and some do neither. Most of the tools have been developed and utilized at sites with unconsolidated geologic media and thus do not apply to fractured media or karst. Indeed, the tools that are available for use in fractured rock systems often provide limited (i.e., point-specific) information because of the high degree of spatial variability at these sites.
At many DNAPL or explosives-contaminated sites, there is inadequate site characterization to support the remediation strategies and success metrics chosen. This is most likely due to unclear objectives, financial constraints, or pressure to show progress and meet deadlines. Despite its technical challenges, some level of source zone characterization is indispensable for the effective management of an environmental remediation effort. Severe overestimation of the source size may inflate the cost of remediation efforts to exorbitant levels. Conversely, missing the source material will jeopardize the success of the cleanup and will require additional characterization and remediation work. The following conclusions and recommendations regarding source characterization are made.
Source characterization should be performed iteratively throughout the cleanup process to identify remedial objectives, metrics for success, and remediation techniques. All sites require some amount of source characterization to support the development and refinement of a site conceptual model. In general, successful source remediation requires information on the nature of the source material, on the site hydrogeology, on the source zone distribution, and on the site biogeochemistry. However, the level of characterization effort required and the tools used at any given site are dependent on site conditions, on the cleanup objectives chosen, and on the technology chosen to achieve those objectives.
An evaluation of the uncertainties associated with the conceptualization of the source strength and location, with the hydrogeologic characteristics of the subsurface, and with the analytical data from sampling is essential for determining the likelihood of achieving success. This is often accomplished through the use of statistical, inverse, and stochastic inverse methods. Unfortunately, quantitative uncertainty analysis is rarely practiced at hazardous waste sites. Obtaining a better handle on uncertainty via increased source characterization would allow eventual remediation to be more precise. It is likely that at most sites, there is not an optimum combination of resources and effort expended on source characterization and thus uncertainty reduction vs. remedial action.
OBJECTIVES FOR SOURCE REMEDIATION
The success of source remediation requires the specification of remedial objectives with clarity and precision. This includes knowing the full range of site
remedial objectives, their relative priorities, and how they are defined operationally as specific metrics. Unfortunately, failure to unambiguously state remedial objectives appears to be a significant barrier to the use of source remediation. Too often, either data presented on the effects of source remediation are irrelevant to the stated objectives of the remedial project, or the objectives are stated so imprecisely that it is impossible to assess whether source remediation contributes to achieving them. For example, the committee is aware of situations in which an explicit operational statement of site objectives, if made prior to beginning source remediation, might have led to a decision not to attempt source remediation.
This widespread problem of vaguely formulated remedial objectives is compounded by the fact that multiple stakeholders at a site not only may have very different objectives, but may also use similar language to describe those very different objectives. Moreover, a particular performance metric may potentially correspond to a variety of different objectives and accordingly be viewed quite differently by different stakeholders. Finally, both the DNAPL problem and the effects of source remediation efforts raise temporal issues that are very poorly addressed by conventional analytical frameworks for assessing risks to human health and the environment.
Chapter 4 describes a variety of objectives possible at sites for which source remediation is a viable option. A distinction is made between absolute objectives, which are important in and of themselves, and functional objectives, which are a means to an end. For example, the objective of reducing contaminant concentrations in groundwater to a specified level at a particular point in time and space may be mandated under a particular regulatory framework as a necessary feature of a successful remediation, in which case it represents an absolute objective. The identical criterion, however, could be selected as a means of ensuring that human health risks have been reduced to an acceptable level. In this case, the objective is functional, because other objectives may achieve a comparable degree of health protection, such as precluding the use of contaminated groundwater.
Physical objectives are discussed first, including mass removal, concentration reduction, mass flux reduction, reduction of source migration potential, plume size reduction, and changes in toxicity or mobility of residuals. Objectives relating to risk reduction, cost minimization, and scheduling are also discussed, many of which have been institutionalized within regulatory, risk assessment, and economic frameworks for site cleanup. The following conclusions and recommendations regarding objectives for source remediation are made.
Remedial objectives should be laid out before deciding to attempt source remediation and selecting a particular technology. The committee observed that remedies are often implemented in the absence of clearly stated objectives, which are necessary to ensure that all stakeholders understand the basis of subsequent remediation decisions. Failure to state objectives in advance virtually guarantees stakeholder dissatisfaction and can lead to expensive and fruitless
“mission creep” as alternative technologies are applied. This step is as important as accurately characterizing source zones at the site.
A clear distinction between functional and absolute objectives is needed to evaluate options. If a given objective is merely a means by which an absolute objective is to be obtained (i.e., it is a functional objective), this should be made clear to all stakeholders. This is particularly important when there are alternative methods under consideration to achieve the absolute objectives, and when it is known or is likely that different stakeholders have a different willingness to substitute objectives for one another.
Each objective should result in a metric; that is, a quantity that can be measured at the particular site in order to evaluate achievement of the objective. Objectives that lack metrics should be further specified in terms of subsidiary functional objectives that do have metrics. Furthermore, although decisions depend upon both technical and nontechnical factors, once a decision has been made, the focus should be on the technical metric to determine if remediation is successful.
Objectives should strive to encompass the long time frames characteristic of many site cleanups that involve DNAPLs. In some existing frameworks, timeframes are very short (rarely longer than 30 years) relative to the persistence of DNAPL (up to centuries), such that alternative actions with significant differences in terms of the speed with which a site can be remedied cannot be distinguished. Within life cycle cost analysis, the chosen timeframes and discount rate can significantly affect cost estimations for different remedies. Decision tools with a more realistic temporal outlook have been developed in other areas of environmental science (e.g., storage and disposal of radioactive materials). Their application to DNAPL problems needs to be considered by the Army and by the site restoration community as a whole.
SOURCE REMEDIATION TECHNOLOGY OPTIONS
Chapter 5 presents those technologies that have surfaced as leading candidates for source zone remediation, including a description of each technology, a discussion of the technology’s strengths and weaknesses, and special considerations for each technology. The discussion of chlorinated solvents focuses on contamination of the saturated zone, as this medium presents the greatest difficulties in terms of site cleanup.
Two technologies commonly used for source remediation function primarily by physically extracting the contaminants from the subsurface. Multiphase extraction employs a vacuum or pump to extract NAPL, vapor, and aqueous phase contaminants, which may then be disposed of or treated. Surfactant and
cosolvent flushing involve introducing a liquid into the subsurface into which the contaminant partitions, and then the mixture is extracted out of the subsurface and is subsequently treated. Two technologies that attempt to transform subsurface contaminants in situ include chemical oxidation and chemical reduction. In both cases, chemicals introduced into the subsurface react with the compounds of concern, leading to their transformation or degradation into less toxic breakdown products. The three most widely applied soil heating methods used for source remediation are steam flooding, thermal conduction heating, and electrical resistance heating—all of which are intended to increase the partitioning of organic chemicals into the vapor or gas phase where they can be extracted under vacuum. In addition, these remedies can achieve destruction of many organic contaminants in situ at sufficiently high temperatures. Two DNAPL remediation technologies either directly or indirectly invoke biological processes to degrade contaminants in situ. Air sparging accomplishes contaminant removal primarily by stripping volatile compounds from the subsurface while simultaneously supporting in situ biodegradation of contaminants. Enhanced bioremediation refers to any in situ treatment in which chemicals are introduced into the subsurface with the goal of stimulating microorganisms that can degrade or transform the contaminants of concern. Although excavation, containment, and pump-and-treat are considered conventional approaches for addressing DNAPL contamination, they are briefly discussed for comparison purposes.
A comparison of the technologies is given in Table 5-7, the goal of which is to help identify a list of the most viable technologies that should be thoroughly evaluated for use under site-specific conditions. The table assesses the types of contaminants for which each technology is suitable and then qualitatively evaluates each technology’s relative potential for mass removal, local aqueous concentration reduction, mass flux reduction, source migration, and changes in toxicity. This evaluation is presented for each of the five hydrogeologic settings described in Chapter 2. The table provides a rank of “high,” “medium,” “low,” or “not applicable” to describe the likelihood that a given technology would be effective at achieving the listed objective. It should be kept in mind that the performance of a given technology is extremely site-specific, as are the objectives associated with any remediation strategy. Thus, the scores are somewhat subjective and should be considered more relative (one technology compared to another) than absolute. Furthermore, a single site may encompass several media settings, or it may not clearly fall into one of the five settings.
The table entries are based, when possible, on reported case studies; more frequently, the entries are based on the committee’s best professional judgment (due to a lack of comprehensive full-scale demonstrations). Thus, few of the metrics in Table 5-7 have been measured in the field for the technologies. Nonetheless, some generalizations can be made. Some source remediation technologies have been demonstrated to achieve substantial mass removal across a range of sites and contaminants. A number of these studies have also demonstrated
concentration reductions (at only one or a few wells), but the meaning of these measurements is highly debatable. Mass flux reduction, reduced migration of the source, and changes in toxicity have not yet been demonstrated at any of the source remediation case studies reviewed. This is partly because of the difficulty in making such measurements. Furthermore, there are few field data to support the hypothesis and existing laboratory data that suggest that partial mass removal can affect local concentration and down gradient mass flux. Thus, available data from field studies do not demonstrate what effect source remediation is likely to have on water quality. The following additional conclusions and recommendations are made regarding technologies for source remediation.
Performance of most technologies is highly dependent on site heterogeneities. In general, the efficiency of flushing methods decreases as the heterogeneity increases, although the degree of impact depends on the specific site characteristics and on the operative processes. In the case of surfactant flushing, foam generated by air injection has emerged as a viable way to mitigate heterogeneities. Steam flushing is affected by preferential flow of the steam, but conduction mitigates this impact to some degree. Soil heating by conduction is least sensitive to heterogeneities because thermal conductivity varies very little with media properties. Chemical oxidation and enhanced bioremediation are more sensitive to heterogeneities than are thermal methods, and air sparging is the most sensitive to heterogeneity because there are no mitigating factors preventing the preferential flow of low-viscosity air and the bypassing of the target DNAPL. Heterogeneities are more likely to affect a technology’s ability to achieve mass removal and local aqueous concentration reductions compared to mass flux from the source zone.
Most of the technologies are not applicable in, are negatively impacted by, or have not been adequately demonstrated in low-permeability or fractured materials. The effectiveness of flushing technologies in low-permeability settings (Type II) is limited due to the difficulty in moving flushing solutions (surfactants, oxidants, reductants, steam) through low-permeability formations. Technologies which do not use fluid flow as a delivery mechanism, such as conductive heating and electrical resistance heating, have greater potential in Type II settings. Applications of source remediation technologies in fractured media (Types IV and V) have been limited due to difficulties in and cost of characterizing the fracture networks and delineating the source zone. In addition, channeling along high-permeability fractures results in poor removal of mass from lower-permeability matrix zones for most technologies, with the possible exception of conductive heating since heat can be conducted efficiently through the rock matrix.
Each technology has the potential to produce negative side effects that need to be accounted for in the design and implementation of that tech-
nology. Examples of potential side effects include surfactant/cosolvent/steam-induced vertical migration of DNAPL, alteration of the redox potential by chemical oxidants or reductants (potentially serving to release previously bound nontarget compounds into the groundwater), and changes in the indigenous microbial population due to chemical or thermal treatment. These side effects can at times be avoided by an experienced design/implementation team. In other cases, the negative side effects should be factored into the design/implementation process.
Almost all of the source remediation technologies evaluated require more systematic field-scale testing to better understand their technical and economic performance. Of the innovative technologies reviewed, only surfactant flooding has amassed a substantial number of field-scale studies in the peer-reviewed literature. Because full-scale applications of source remediation technologies are scarce, there is insufficient information to thoroughly evaluate most technologies, especially with regard to the long-term impact of mass reduction in the source zone. Furthermore, due to insufficient economic data and the site-specific nature of both performance and cost, it is not possible to generically predict the impact of source remediation technologies on life cycle costs.
The level and type of source zone characterization required to design, implement, and monitor the performance of remedies is dependent on the chosen objectives and the remediation technology. For example, in situ chemical oxidation requires accurate estimates of source zone mass and composition and matrix oxygen demand, or else the remedy could be plagued by stoichiometric limitations or by the consumption of oxidant by unidentified co-contaminants. The properties of the source material (e.g., composition, viscosity, density, interfacial tension) should be determined for field-weathered samples in order to assess such remedies as surfactant-enhanced flushing. The location and geometry of source zone materials should be known to some level of certainty in order to design containment systems. For example, the most effectively designed slurry wall will have less effect on downstream mass flux if it is placed across the source zone rather than around it. With respect to performance monitoring, judging the effectiveness of in situ chemical oxidation by monitoring mineralization products or by monitoring the consumption of oxidant could overestimate treatment effectiveness in cases where alternate contaminants are present.
Development of treatment technologies for explosives source zones is in its infancy because the characterization of explosive source materials and of their interactions with geologic media lags far behind the knowledge base that exists for DNAPLs. Before one can understand the utility or performance characteristics of treatment technologies for explosives contamination, one should understand the chemical and physical nature of the explosives source zones. Furthermore, source areas containing high concentrations of explosives have the
potential for dangerous explosions during remediation, which will necessitate laboratory and field assessment of explosives source zones within specialized facilities.
ELEMENTS OF A DECISION PROTOCOL FOR SOURCE REMEDIATION
Investments in source remediation technologies have often failed to achieve the desired reductions in risk and/or site care requirements, partly because historical releases of DNAPLs and explosives are technically difficult to clean up, but also because of how source zones are managed. The design and implementation of a successful source remediation project involve the iterative characterization of the source zone, development of remediation objectives, and evaluation of technologies—a process that is sufficiently complex to warrant a formal protocol. Chapter 6 describes the elements of a protocol to assist project managers in designing, implementing, and assessing the effects of source remediation. These elements are laid out in Figure ES-1, which depicts a six-step process that includes activities (white boxes), data and information collection (gray boxes), and decision points (gray diamonds). The six steps are taken sequentially as source remediation moves forward. As can be seen from the figure, however, there may be multiple iterations of each step until a decision can be made to proceed to the next step.
Each of these six steps is described in detail, using a hypothetical example of a typical hazardous waste site and drawing on information from previous chapters. Steps 2 and 3 in Figure ES-1 focus on identifying absolute and functional objectives that are clearly articulated and verifiable (one of the distinguishing features of the framework). The figure emphasizes the role of managing data gaps and uncertainty via site-specific data collection (as exemplified by “collect data and refine site conceptual model” shown in gray boxes). As discussed in Chapters 2 and 3, considerable uncertainty exists at almost every hazardous waste site with respect to the location and extent of contamination. The protocol focuses users on recognizing the limitations of their current understanding, on the importance of collecting the necessary information to effectively make decisions, and on managing plausible variations from perceived conditions. Step 4 in Figure ES-1 involves referring back to the comparison table of technologies in Chapter 5 (Table 5-7) to determine which technologies might be viable given the contaminant type present, the hydrogeologic setting, and the chosen functional objectives. Collection of adequate data to characterize the source, development of clear absolute and functional objectives and their metrics, and remedy evaluation have seldom been done at the level described in this report. If all of these steps are not included, source remediation at an individual site will have a low probability of success.
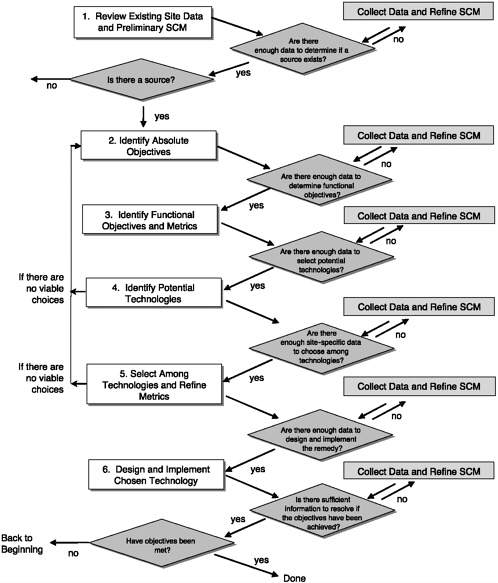
FIGURE ES-1 Six-step process for source remediation. SCM = site conceptual model.
The Army should develop and use a detailed protocol consistent with the elements prescribed in Figure ES-1. A protocol specific to source zones is needed to aid stakeholders in optimizing the benefits derived from investments in remediating source zones. The key attributes that need to be addressed are pursuing actions that effect intended changes, understanding the extent to which objectives are attainable, and being able to measure progress toward desired
objectives. The protocol will need to be integrated into the existing remedy selection frameworks used by the Army at individual sites, including Superfund, RCRA, relevant state laws, or the Base Realignment and Closure program.
Involvement of potentially affected parties is essential to the success of source remediation. Stakeholder participation is needed to better understand the range of absolute objectives at a given site, to develop functional objectives, and to gain consensus on appropriate actions. Without adequate public participation, critical elements of solutions may be missed, a subset of the involved parties may feel that their needs have been ignored, and/or false expectations may develop as to what can be achieved. As for all relevant stakeholders, knowledge acquisition by the public is essential to making decisions about source remediation.
With respect to the future use of source removal as a cleanup strategy, an important conclusion that can be made from reviewing source zone remediation attempts to date is that the data are inadequate to determine how effective most technologies will be in anything except the simpler hydrogeologic settings. Furthermore, it is unlikely that available source remediation technologies will work in the most hydrogeologically complex settings such as karst. Beyond defining these extremes, it is difficult to make generic statements about source remediation, as most field studies have not provided quantitative information on the ability of the technologies to meet most remediation objectives. In a few carefully documented cases, removal or destruction of a large fraction of the contaminant mass present in the source zone was achieved. There have also been several well documented remediation projects in which concentrations in monitoring wells were reduced to a small fraction of pre-project concentrations, although few of these cases provided long-term data that might reveal rebound. Almost none of the dozens of projects reviewed by the committee contained quantitative mass flux measurements.
Despite these drawbacks, by following the elements of a source remediation protocol illustrated in Figure ES-1, project managers will be able to make critical decisions regarding whether and how to attempt source remediation and thereby accomplish a more beneficial distribution of resources. The steps presented in Figure ES-1—determining whether a source exists; developing absolute and functional objectives and their metrics; selecting, designing, and implementing a technology; and collecting data to support all these decisions—have seldom been conducted in the manner described in this report. Not following these steps has led to source remediation technologies being prematurely scaled up at poorly characterized sites, at sites where there is known complex hydrogeology, and where there is no clear reason for proceeding with the project.
Finally, several technologies show enough promise, in terms of demonstrated mass removal and concentration reduction in simple hydrogeologic
settings, to warrant further investigation to determine their long-term effects on water quality, especially if objectives such as mass flux reduction become more prevalent. Thus, future work should attempt to determine the full range of conditions under which these technologies can be successfully applied, and to better understand how mass removal via these technologies affects water quality.