The Use of Sodium-Cooled Fast Reactors for Effectively Reprocessing Plutonium and Minor Actinides*
V. I. Matveev, V. A. Yeliseev, and Ye. V. Poplavskaya
State Scientific Center Institute of Physics and Power Engineering
The most urgent problem associated with nuclear power industry operations is that of the accumulation of high-level radioactive wastes in the form of fission products and actinides. The latter are defined as plutonium and the so-called minor actinides—neptunium (Np), americium (Am), and curium (Cm). The water-moderated water-cooled power reactor (VVER-1000), for example, produces about 20 metric tons of spent fuel annually, with this material containing approximately 1 percent plutonium (about 200 kilograms) and about 0.1 percent minor actinides (about 20 kilograms). If you consider the fact that the capacity of all the atomic power stations in Russia is on the order of 22 Gwt, then the nuclear power industry produces approximately 500 metric tons of wastes annually, including about 10 metric tons of plutonium and about 0.5 metric tons of minor actinides.
To date, studies have been made at the conceptual level regarding various means of using reactor technology to recycle wastes from the nuclear power industry. These studies primarily focus on fast reactors, although VVER-type thermal reactors, for instance, might also be used for the effective reutilization of plutonium.
The most important problem lies in recycling minor actinides. Plutonium may be used as an efficient nuclear fuel in thermal and fast reactors to produce electricity. It is impossible to use minor actinides (Am, Np, Cm) for these purposes in thermal reactors, and their constant accumulation as a result of nuclear
power operations creates a significant environmental threat. Minor actinides may be reprocessed as a side function in fast oxide-fueled reactors. However, this method is not sufficiently effective. A more efficient method involves burning minor actinides in specialized cores using fuel that does not contain uranium-238. Including fast reactors with such cores in the nuclear power industry’s closed fuel cycle would make it possible to stabilize the accumulation of minor actinides. In such a system all actinides produced by the industry are also recycled by it, and only the portion of these materials that cannot be separated out through chemical reprocessing must be stored as wastes. Organizing such a system, however, requires major capital investments for the creation of a large number of external fuel cycle enterprises. Therefore, the burning of plutonium and minor actinides in the open fuel cycle is of interest.
This report considers various means of using sodium-cooled fast reactors for reprocessing plutonium and minor actinides produced in nuclear power industry operations.
THE CONCEPT OF FAST REACTORS AS ACTINIDE BURNERS
In analyzing the possible use of BN-type fast reactors1 to recycle wastes from the nuclear power industry, let us highlight two problems: reprocessing of plutonium and reprocessing of minor actinides.
Efficient recycling (burning) of plutonium can be carried out in mixed-oxide-fueled fast reactors with reduced internal breeding due to increased fuel enrichment, but the use of mixed oxide (MOX) fuel for these purposes is not the best option. From the standpoint of secondary plutonium output, it would make sense to consider fuel compositions without uranium-238, as this isotope is the source that produces both plutonium and other very environmentally harmful actinides. Fuel without uranium-238, with this isotope being replaced by some inert matrix, is of great interest in this regard. A number of technological laboratories in Russia, France, and Japan have been working recently to develop such a fuel. For example, a power reactor (fast or thermal) could burn approximately 750–850 kg/GW (el) of plutonium per year without producing any secondary plutonium. Burning plutonium in reactors using fuel without uranium-238 is much more efficient in comparison with using MOX fuel.
Another important problem involves the reprocessing of minor actinides. Plutonium may be used as an efficient fuel in thermal and fast reactors for electricity production purposes. Minor actinides cannot be used in thermal reactors for these purposes, and their continual accumulation as a result of nuclear power industry operations creates a significant environmental threat. Let us note once again that plutonium from spent fuel from thermal reactors contains about 7 percent minor actinides, an amount that increases over time as a result of the decay of plutonium-241 (T1/2 = 14.7 years) and its transformation into americium-
241. Because the activity and radioactive danger of minor actinides are substantially (about three times) greater than the analogous characteristics for plutonium, reprocessing only plutonium will not in and of itself resolve the problem of the environmental safety of nuclear wastes. To resolve this problem we might also use fast reactors with fuel not containing uranium-238. It should be noted that the reprocessing of minor actinides could be carried out only as a side function in oxide-fueled fast reactors. Here, in assessing the possible (permissible) amount of minor actinides that could be included in the fuel, it is essential to take into account the nuclear safety of the reactor, one of the main criteria of which is the sodium void reactivity effect (SVRE). For example, assessments made for the BN-800 reactor demonstrate that it would be possible to burn approximately 20 kilograms of minor actinides over the course of a year. At this rate the fast reactor could serve one VVER-1000 reactor. However, to this end it would be necessary to significantly expand the number of fast reactors in the nuclear power sector—up to a level totaling 50 percent of all operating reactors. Meanwhile, about 100 kilograms of minor actinides per year could be burned in specialized cores in BN-800-type fast reactors using fuel without uranium-238, replaced instead with an inert matrix. In the long term it may be possible to burn minor actinides more efficiently in subcritical reactors using accelerators (an accelerator-driven system). In such a system it would be possible to burn up to 250 kilograms of minor actinides per year using an 800-MWt (thermal) fast-neutron subcritical reactor.
Using fuel without uranium-238 in BN-type fast reactors and in advanced subcritical fast reactors opens up real possibilities for eliminating high-level and long-lived wastes from nuclear power production.
USING BN-800-TYPE REACTORS WITH FUEL WITHOUT URANIUM-238 TO BURN ACTINIDES
To date, a rather broad range of studies has already been conducted to analyze and select various fuel compositions without uranium-238, with this isotope being replaced by an inert matrix. Compositions based on zirconium carbide, aluminum nitride, magnesium oxide, and other compounds are being considered. Current research studies have focused on a composition based on magnesium oxide.
The core using fuel without uranium-238 has a number of special characteristics. First of all, excluding uranium-238 ensures that the SVRE will have a negative value, which is an undoubted advantage of such active zones. Meanwhile, there is a noticeable reduction in the magnitude of the Doppler effect, which in traditional cores is determined by uranium-238. The Doppler effect could be partially restored by introducing resonance absorbers into the fuel composition, with the best of these being iron, niobium, and tungsten. Excluding
TABLE 1 Basic Characteristics of a BN-800 Reactor Using Fuel Without Uranium-238
Characteristic |
Value |
Nominal power, MWt (electrical) |
800 |
Number of fuel rods in active zone |
565 |
First subzone |
145 |
Second subzone |
420 |
Fuel composition |
(Pu, MA)O2 MgO |
Effective fuel density, g/cm3 |
4.5 |
Actinide content (Pu, MA) in fresh fuel, g/cm3 |
1.6 |
SVRE in core, %Δk/k |
~0 |
Doppler effect in active zone, %Δk/k |
−0.235 |
Rate of reactivity change, %Δk/k/month |
3.5 |
Time between reloadings, in months |
3.0 |
Maximum fuel pin linear power Wt/cm |
500 |
TABLE 2 Quantity of Actinides Produced in Spent Fuel from Thermal Reactors, kg/year/GW (electrical)
Isotope |
Uranium Fuel |
Uranium-Plutonium Fuel (30%) |
All |
235 |
385 |
Pu238 |
2.8 |
6.8 |
Pu239 |
121.7 |
160.1 |
Pu240 |
53.2 |
96.7 |
Pu241 |
27.5 |
56.9 |
Pu242 |
12.8 |
31.8 |
Np237 |
7.1 |
11.2 |
Am241 |
6.4 |
8 |
Am242 |
0.006 |
0.03 |
Am243 |
2.6 |
9.1 |
Cm242 |
~0a |
0.05 |
Cm244 |
1 |
4 |
Cm245 |
~0b |
0.2 |
aApproximately 0, due to decay. bThe number is too small to value. |
uranium-238 also leads to a substantial increase in burn-up reactivity rates, which in turn leads to a decrease in the interval between reloadings. However, the most painful shortcoming of such cores is the great difference in power of fresh and spent fuel assemblies, which significantly increases the power inequality. In developing a core with fuel without uranium-238 for the BN-800 reactor, the latter of the above-mentioned problems was resolved as follows. The core, consisting
of two subzones having different plutonium contents (analogous to the zones of low and high enrichment in traditional reactors), is separated into six concentric subzones (three subzones in each of the subzones with different plutonium contents), and reloading is carried out through the internal subzones by means of shifting fuel subassemblies from the periphery to the center, thus forming an equalized power field. In this process the equalization is optimized with a fuel burn-up of approximately 35 percent. It is possible to increase the fuel burn-up, but this will increase the inequality of the power field and will require the core to have a greater volume. The basic characteristics of the BN-800 reactor using fuel without uranium-238 are presented in Table 1.
These characteristics could be improved through the selection of a core for the specialized reactor. The core option cited was selected on the basis of the existing limitations of the BN-800 reactor, for example, the number of control rods and scram system and the volume of the core.
A BN-800 reactor with the above-described core can effectively fulfill the function of a reactor-burner of plutonium and minor actinides. Here we will consider a simple model for the operation of a nuclear power system consisting of VVER-1000 thermal reactors and BN-800-type fast reactors. In this model the BN-800 reactors use plutonium as well as minor actinides (7 percent), which are extracted from the spent fuel from the VVER reactors and which are mixed with plutonium and minor actinides extracted from the spent fuel from the BN-800 reactor. Table 2 shows the actinide content of the spent fuel from the VVER-type thermal reactors.
The dynamics of changes in fuel cycle characteristics have been studied for
TABLE 3 Established Isotope Composition of Fresh Fuel for Fast Reactors (kg/t)
Isotope |
Touter = 1 Year |
Touter = 3 Years |
Pu238 |
46.6 |
53.5 |
Pu239 |
249 |
250.1 |
Pu240 |
358.7 |
358.3 |
Pu241 |
80.2 |
67.9 |
Pu242 |
118.6 |
119 |
Np237 |
17.1 |
17.8 |
Am241 |
47.2 |
62.1 |
Am242 |
3 |
3.5 |
Am243 |
46.6 |
43.7 |
Cm242 |
0.6 |
0.06 |
Cm244 |
28.3 |
20.1 |
Cm245 |
5.3 |
4 |
two periods of cooling of spent fuel from fast reactors, Touter = 1 year and Touter = 3 years. Calculations have shown that during the process of recirculation, the content of minor actinides in the fuel increases steadily, from approximately 7 percent (initial loading of fuel from the VVER) to 10–13 percent in quasiequilibrium mode. This mode is established after about 25 cycles. The calculated characteristics of the fuel in quasiequilibrium mode are presented in Table 3.
In the nuclear power model under consideration one BN-800-type reactor using fuel without uranium-238 could reprocess the plutonium and minor actinides produced in 1.8 VVER-1000 reactors.
RECYCLING MINOR ACTINIDES IN A BN-800-TYPE REACTOR
The previously discussed study has shown that by repeatedly recirculating plutonium fuel containing 7 percent minor actinides through the core of a BN-800 reactor using fuel without uranium-238, it is possible to burn approximately 36 kilograms of minor actinides per year, thus processing the output of 1.8 VVER-1000 reactors. The amount of minor actinides increases in a stable manner toward a state of equilibrium at the level of about 12 percent.
How much might we increase the minor actinide content in fresh plutonium fuel to be loaded into a BN-800 reactor? The answer to this question depends on the results of studies of many factors, including the radiation characteristics and heat release characteristics of the fresh fuel, which have a substantial impact on the organization of the technological process involved in manufacturing the fuel. Here we note only the most important physical factor, namely, the magnitude of the SVRE. Calculations indicate that for this sort of core design, the permissible level of minor actinide content in fuel is approximately 15 percent if the goal is to ensure a zero SVRE value in the active zone. We shall also note that here the reserve SVRE provided by the sodium cavity is not considered.
Research on the dynamics of the fuel cycle given 15 percent minor actinide content in the fresh fuel indicates that the level of minor actinides in quasiequilibrium status will total approximately 18 percent. Setting up the fuel cycle in such a manner makes it possible to recycle about 1.5 times more minor actinides per year than if the fuel cycle included fuel with 7 percent minor actinide content, meaning that about 55 kilograms of minor actinides would be reprocessed per year.
Increasing the efficiency of minor actinide burning is possible by using fuel based on uranium-235. Calculations indicate that when using this type of fuel, a zero value for SVRE is maintained when adding 35 percent minor actinides. In this case minor actinide burning efficiency is increased by 60 percent over the preceding case and will total about 90 kilograms per year. Thus, the core of a BN-800 reactor using uranium-235-based fuel could serve six or seven VVER-1000 reactors.
It is interesting to note that in quasiequilibrium mode, the proportion of
TABLE 4 Calculated Composition of Unloaded Fuel in Reactors Using Fuel Feed Based on Plutonium and Uranium-235 (Relative Percentages)
Isotopes |
Fuel |
|
85% Pu, 15% MA |
65% U235, 35% MA |
|
U234 |
0.18 |
2.90 |
U235 |
0a |
36.96 |
U236 |
0a |
29.99 |
Np237 |
7.23 |
10.11 |
Pu238 |
4.65 |
8.62 |
Pu239 |
41.57 |
1.67 |
Pu240 |
24.09 |
2.35 |
Pu241 |
7.41 |
0.26 |
Pu242 |
5.48 |
0.81 |
Am241 |
6.39 |
2.88 |
Am242 |
0.19 |
0.15 |
Am243 |
2.13 |
1.94 |
Cm243 |
0.03 |
0.01 |
Cm244 |
0.58 |
1.00 |
Cm245 |
0.05 |
0.15 |
Cm246 |
~0b |
0.07 |
aThis isotope was absent in this fuel composition. bThe number is too small to value. |
minor actinides is reduced to approximately 18 percent. Table 4 presents the results of calculations by composition of loaded fuel for reactors using two types of fuel, one containing 85 percent plutonium and 15 percent minor actinides, the other containing 65 percent uranium-235 and 35 percent minor actinides. It may be noted that the plutonium composition that arises when the uranium-235 fuel is used includes a large amount of plutonium-238.
FAST REACTORS AS BURNERS OPERATING IN THE OPEN FUEL CYCLE
In developing cores for fast burner reactors it is essential to select fuel compositions that would ensure the high stability of the spent fuel during its subsequent burial for storage. A number of Japanese researchers have developed a proposal for using highly stable practically insoluble rock-like fuel (ROX fuel) in thermal reactors for the efficient reprocessing of plutonium. It would seem expedient to use this sort of fuel in actinide-burning fast reactors as well.
In developing ROX-fueled cores for operation in the open fuel cycle, one of
the main criteria of efficiency is the achievement of maximum possible fuel burn-up, but maximum burn-up is limited by a number of factors. There is a purely physical limitation associated with the fact that increasing the fuel burnup requires increasing the load of fissile materials in the fresh fuel. The properties of ROX fuel are maintained when the content of fissile materials in the matrix is no more than 40 percent, that is, maximum loading by fission materials should not exceed 2.4 grams per cubic centimeter (initial density of ROX-fuel is approximately 6.0 grams per cubic centimeter). Another limitation associated with the operating capacity of the engineering materials (fuel subassembly and fuel rod casings) occurs during fast neutron irradiation: a permissible neutron flux on the order of 200 displacement per atom (dpa) may be taken as a preliminary norm.
The Institute for Physics and Power Engineering has conducted studies on the efficiency of burning plutonium and minor actinides in a fast BN-800-type reactor fully loaded with ROX fuel. The following ROX-fuel composition was used in the study:

Various possible ratios between the fuel and the inert matrix were considered. Special parametric studies showed that the greatest burning in the active zone could be achieved by using fuel based on pure plutonium dioxide (without a matrix).
Power in this core is equalized by repositioning the fuel subassemblies (SA) during reloading.
As a result it is possible to achieve an average along the height of SA burnup of approximately 83 percent of unloaded power plutonium fuel in the core. The maximum burn-up in the central plane totals about 88 percent. The same assessment for the burning of weapons-grade plutonium shows an average burnup per fuel rod of about 88 percent and a maximum of 92 percent. The burn-up rate for the main isotope, plutonium-239, is 97 percent. These are the maximum burn-up levels possible in principle due to the physics of fast reactors.
The option for the core based on pure plutonium dioxide cannot be technically feasible first due to the very high irradiation integral for the engineered materials (more than 1000 dpa) and second because its fuel must be pure plutonium dioxide, not a ROX composition. Therefore, the following aspects were taken into account during further development work on a ROX-fueled core:
-
The requirements of deep and fast fuel burn-up are mutually exclusive. Basic parameters for the core should be selected on the basis of the conditions of technical feasibility for the core.
-
The maximum permissible limit for radiation damage to engineered materials is defined as 200 dpa. This condition in fact clearly defines the permissible fuel burn-up, including the fuel-matrix ratio and the thickness of this composition.
-
The fuel composition must have ROX properties, that is, it must be suitable for direct burial in geologic formations. The maximum plutonium content with which the composition maintains its rock-like (ROX) properties is 40–45 percent (by weight). This is an additional condition that determines the selection of a possible fuel composition.
Studies on the selection of a composition that would fulfill the above-listed restrictions have shown that the composition must have a density (for plutonium dioxide) of 2.61 grams per cubic centimeter and consist of 42 percent PuO2 and 58 percent MgAl2O4. The density of the fuel composition itself will be about 6.22 grams per cubic centimeter.
For comparison purposes Table 5 presents the basic characteristics of three different types of active zones. The first is the so-called designed option, all of the parameters of which are included in full in the original design for the BN-800 reactor.2 The second is the maximum option, in which the maximum fuel burnup is achieved, and the third is the optimal or technically feasible option.
One can see that the spectrum of characteristic changes is very broad. In the maximum option the neutron spectrum is very hard (since there is no uranium-238, which is a moderator of neutrons); therefore, this option features a large positive SVRE value due to fission of threshold isotopes, and the Doppler effect is very low (due to the very low proportion of intermediate neutrons, which determine this effect). In the initial option the inert matrix substantially mitigates the spectrum, and a significant proportion of intermediate neutrons appears, as a result of which the SVRE goes from a positive to a negative value and the Doppler effect increases by almost two orders of magnitude.
In order to increase fuel burn-up further the idea arose to use radial leakage neutrons. To accomplish this a post burn zone of burned SA is placed around the
TABLE 5 Calculated Range of Changes of Basic Characteristics of a Core Burner
Option |
Designed |
Maximum |
Optimal |
Fuel density (for plutonium dioxide), g/cm3 |
1.05 |
8.6 |
2.61 |
Average (maximum) fuel burn-up, % heavy atoms (h.a.) |
30 (36) |
83 (88) |
50 (58) |
Ratio of power in fresh/spent fuel |
1.97 |
8.4 |
2.8 |
Radial power inequality, Kr |
1.78 |
3.24 |
2.21 |
Vertical power inequality, Kz |
1.28 |
1.25 |
1.14 |
Volume power inequality r, Kv |
2.28 |
3.73 |
2.52 |
Proportion of power in the core/post burn zone, % |
—a |
93/7 |
93/7 |
Rate of reactivity drop (over 30 days), %Δk/k |
4.27 |
0.77 |
1.77 |
SVRE of core, %Δk/k |
−1.6 |
+1.9 |
+0.07 |
Doppler effect = Δk/k/ln(T-1500/T0-1500), %Δk/k |
−0.0112 |
−0.00048 |
−0.0068 |
aPost burn zone is absent in this core option. |
TABLE 6 Calculated Values of Average Burn-up Depth of Unloaded Fuel, Percent Total Activity (Numerator: From Core; Denominator: From Post Burn Zone)
Option |
Designed |
Maximum |
Optimal |
Post burn zone without moderator |
|
|
|
Post burn zone with moderator (zirconium hydride) |
|
|
|
core (the volume of the former was set as equal to the volume of the core itself, and the additional burning time of the fuel in it equaled the time of core lifetime). Inasmuch as this zone has a weak effect on reactor criticality, any radical changes in the neutron spectrum, for example, making a fast reactor into a thermal one by adding a moderator to the periphery zone, are possible without damaging the core. Table 6 provides burn-up data for the three previously selected options, taking into account the additional burning of fuel on the periphery.
The optimal option, which meets all technical limitations, produces a burnup in unloaded fuel of about 55–60 percent h.a., with burn efficiency in the periphery zone, in this case being somewhat higher than in the maximum option, as the moderator somewhat increases this efficiency rate.
STUDY OF THE POSSIBILITY OF DEEP BURNING OF MINOR ACTINIDES IN THE CORE OF A BN-800 REACTOR NOT FULLY LOADED WITH FUEL WITHOUT URANIUM-238
This study of the burning of minor actinides used a calculated model of an expanded core in a BN-800 reactor (702 fuel assemblies in the core and 30 control and scram system rods). The fuel subassemblies in this model were loaded with common MOX fuel, but part of the fuel subassemblies were replaced with burn subassemblies containing only minor actinides (AmO2 and NpO2) in an inert matrix (spinel Al2MgO4) or in a moderator (zirconium hydride). At this stage the placement of these materials was considered homogeneous. The quantity of plutonium or minor actinides in the burn subassemblies varied across a very wide range—from 100 percent (about 25 kilograms per subassembly) to 0.1 percent (25 grams per subassembly). These subassemblies were placed in regular order throughout the entire core. The total quantity of these burn subassemblies in different core options varied from 84 to 137. This theoretical research produced the following correlations between the burn-up for plutonium and minor actinides and the composition of the burn subassemblies over time as presented in Table 7 and Figure 1.
As shown in Table 7 and Figure 1, increasing the loading of minor actinides in the fuel elements of the fuel subassemblies being burned leads to a small reduction in the fuel burn-up. This is associated with a certain reduction in minor actinide cross-sections as a result of the increase in resonance blocking.
The introduction of a strong moderator into the fuel subassemblies leads to a sharp increase in the burn-up of minor actinides (up to approximately 95 percent h.a. if minor actinide [MA] content is low). This is because of the fact that in the presence of a strong moderator the neutron spectrum becomes close to thermal, in which the cross-sections of nuclide interaction increases by hundreds of times.
This research allows us to make the following conclusions:
-
It is possible to achieve deep burn-up rates for minor actinides (more than 90 percent h.a.) in a relatively short period of time (that is, in one or two fuel lifetimes), but only with a strong moderator and comparatively small quantities of loaded minor actinides (about 2–3 kilograms of minor actinides per fuel SA).
-
The efficient burning of minor actinides in a strong moderator is accompanied by noticeable flashes of power at the borders of neighboring fuel subassemblies. In order to suppress this phenomenon one might use, for example, athin (0.2–0.5 millimeter) layer of cadmium to cover the fuel subassembly wrapping.
TABLE 7 Calculated Values for Burn-up (Percentage) for Americium and Neptunium Depending on Content and Use of a Matrix or Moderator over 800 Days (Figures in Parentheses over 400 Days)
Quantity of MA in Fuel Composition, Percentage |
Mass of MA in Fuel Rods, kg |
Burn-up, Percentage, h.a. |
|||
Americium + Matrix |
Americium + Moderator |
Neptunium + Matrix |
Neptunium + Moderator |
||
0.1 |
0.025 |
25.2 (11.0) |
98.6 (88.3) |
24.1 (10.5) |
99.4 (94.3) |
0.5 |
0.125 |
24.7 (10.8) |
98.4 (86.1) |
23.3 (10.2) |
99.4 (93.9) |
1 |
0.25 |
24.3 (10.7) |
98.2 (84.3) |
23.1 (10.1) |
99.3 (93.1) |
5 |
1.25 |
23.1 (10.1) |
92.8 (62.3) |
22.2 (9.8) |
96.9 (78.2) |
10 |
2.5 |
22.1 (9.7) |
79.1 (44) |
21.5 (9.4) |
91.1 (58.1) |
20 |
5 |
20.8 (9.1) |
56.8 (28) |
20 (9) |
65.3 (32.1) |
30 |
7.5 |
19.8 |
42.8 |
19.2 |
43.9 |
50 |
12.5 |
18.7 |
29.3 |
18.3 |
25.6 |
70 |
17.5 |
17.9 |
22.3 |
17.7 |
20.1 |
80 |
20 |
17.6 |
20.1 |
16.8 |
18.8 |
90 |
22.5 |
17.4 |
18.1 |
17.4 |
17.9 |
100 |
25 |
17 |
16.9 |
17.3 |
17.1 |
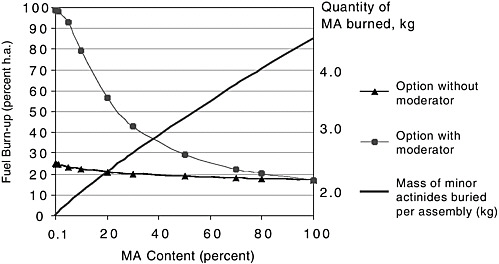
FIGURE 1 Dependence of fuel burn-up and mass of burned minor actinides (per burn subassembly for matrix option) on content of minor actinides in matrix (moderator) (time of irradiation taken as equal to two reactor lifetimes or 2 × 400 days).
-
The duration of burning the above-mentioned materials in a moderator could be either 400 or 800 days, and in the latter case the level of radiation damage to the casings of the burn subassemblies will be somewhat greater than 90 dpa. This is fully permissible if existing EP-450-type steels are used.
Preliminary research has shown that for destroying minor actinides, a core not fully loaded with fuel based on an inert matrix is highly efficient. This sort of core makes it possible in real time (no more than three years) to achieve a minor actinide burn-up rate of more than 90 percent by total activity. This burning is carried out in special burn subassemblies loaded with elements containing zirconium hydride as well as other elements containing minor actinides in an inert matrix. When 84 such burn subassemblies are installed in the expanded core of a BN-800 reactor using oxide fuel, it is possible to burn about 65 kilograms of americium or neptunium per year. This amount could be increased by approximately 50 percent (up to 100 kilograms) if the number of burn subassemblies is increased to about 137. This would make it possible to recycle the minor actinides produced in about five VVER-1000 reactors.
CONCLUSION
The results cited in this paper illustrate the great usefulness of continuing and developing scientific research and design efforts in the area of fast sodium
reactors for use as actinide burners, including materials science and engineering research efforts focusing on fuel compositions without uranium-238, with that isotope being replaced by an inert matrix. Inasmuch as the selection of and justification for the use of this or that fuel composition depend substantially on the design of the fast burner reactor and its fuel cycle (for example, an open fuel cycle with subsequent burial of spent fuel, a closed fuel cycle with reprocessing of spent fuel, and so forth), technological research must be conducted along with conceptual research on the selection of fast burner reactors and their fuel cycles as well as on the scientific justification for this selection.
REFERENCES
Akie, H., T. Muromura, H. Takano, and S. Matsuura. 1994. A new fuel material for once-through weapons plutonium burning. Nuclear Technology 107:189–182.
Byburin, G. G., I. Yu. Krivitski, A. P. Ivanov, V. I. Matveev, and E. V. Matveeva. 1995. Plutonium burning in fast reactor cores using unconventional fuel without U-238. Unconventional options for plutonium disposition . IAEA, November 7–11, 1994, Obninsk, Russian Federation. IAEA-TECDOC-840, pp. 219–228.
Krivitski, I. Yu., M. F. Vorotyntsev, L. V. Korobeinikova, and V. K. Pyshin. 1998. Evaluation of self-protection of fast reactor core with uranium free core. Transactions of ENC ’98 Congress, Nice, France, October 25–28, 1998. 4:100–104.
Krivitski, I. Yu. 1999. Actinide and fission product burning in fast reactors with a moderator. Proceedings of the International Conference on Future Nuclear Systems, GLOBAL ’99, United States, August 29–September 3, 1999. Jackson Hole, Wyoming.
Matveev, V. I., A. P. Ivanov, and E. V. Efimenko. 1992. Use of fast reactors for actinide transmutation. A Concept of Specialized Fast Reactor for Minor Actinide Burning. IAEA, September 22–24, Obninsk, Russian Federation.
Matveev, V. I., I. Yu. Krivitski, and A. G. Tsikunov. 1996. Concept of fast reactors—plutonium burners and their fuel cycle. Proceedings of the International Conference on the Physics of Reactors, PHYSOR ’96, Mito, Japan, September 16–20, 1996. 3:p. 21.
Matveev, V. I., I. Yu. Krivitski, V. A. Elissev, and A. L. Kochetkov. 1999. The role of fast reactors in utilization of long-lived nuclear wastes. Proceedings of the International Conference on Future Nuclear Systems, GLOBAL ’99, United States, August 29–September 3, 1999. Jackson Hole, Wyoming.
Matveev, V. I., A. G. Tsikunov, and I. Yu. Krivitski. 1999. Nuclear power systems using fast reactors to reduce long-lived wastes. Pp.179–199 in Safety Issues Associated with Plutonium Involvement in the Nuclear Fuel Cycle, eds. T. A. Parish et al. Dordrecht: Kluwer.
Muromura, T., et al. 1995. Unconventional options for plutonium disposition IAEA-TECDOC-840, pp. 253–262.
Poplavsky, V. M., V. I. Matveev, V. A. Rogov, et al. 2001. Physical and engineering problems of increasing the safety and efficiency of actinide burning in a cutting-edge fast reactor. Monograph, Obninsk, Russian Federation. [in Russian]