7
Life Detection and Biomarkers
Prior chapters suggest some constraints on where carbon-based life might be found. In particular, environments that have a temperature greater than 500 K, lack a liquid solvent, and lack carbon are not likely locales for life related to the type we are familiar with on Earth. Not discussed above, however, are simple approaches to distinguishing between the products of Darwinian evolution and the products of inanimate processes. Even if organic molecules are necessary for life, their presence is clearly not a sufficient condition for the existence of life. Organic compounds are abundant in the cosmos, as meteorites show.
7.1
CHIRALITY AS A BIOMARKER
The vast preponderance of biologically formed chiral compounds are synthesized exclusively as one or other enantiomer. Enantiomers are isomers for which the left- and right-handed forms have the same physical behavior but respond differently in chemical reactions with other chiral molecules. See Box 7.1 for an explanation of stereoisomerism. In chemical processes, left- and right-handed molecules are generally formed in equal amounts, unless chiral reagents, catalysts, or surfaces are present to introduce a bias. Also, some organisms may synthesize the same chiral compound in different enantiomeric forms (e.g., bacterial peptide toxins). For the formation of regular structures when these monomers are assembled, however, it is generally more efficient to have the same handedness in all molecules. Thus, 100 percent of amino acids in terran proteins have the same absolute spatial relationship of the amino group, the acid group, and the side chain distinctive for the amino acid and are all designated “L”. Likewise, all of the sugars in nucleic acids have the opposite spatial relationship and are designated “D”. Enantiomeric excess (also called chirality, or handedness), the predominance of one enantiomer over the other for a given assembly of atoms, is therefore believed to be a universal feature of chemistry characteristic of living systems.
Once an organism dies and its biochemicals are released into the environment, their chiral purity (and optical activity) may or may not persist depending on the relative chemical stability of the bonds in the vicinity of the chiral center. Various natural chemical processes can lead to racemization, the formation of mixtures of the two enantiomers. While racemization may result in loss or corruption of a biological signature, the rate at which it happens can also have a practical application. The best known example is the dating of fossil organic matter on the basis of the degree of amino acid racemization.
BOX 7.1 Stereoisomerism Molecules are three-dimensional. A carbon atom with four single bonds lies at the center of a tetrahedron. The atoms to which the carbon is bonded are at the vertices of the tetrahedron. Those atoms are in turn likely to be bonded to other atoms. If the chemical structures at the four corners all differ, however slightly, the mirror images of the tetrahedron will not be superimposable (Figure 7.1.1). Mirror-image stereoisomers that are not superimposable are called enantiomers; stereoisomers that are not enantiomers are called diastereomers. Enantiomers possess chirality, or handedness, and when dissolved rotate the plane of polarized light when it is passed through the solution. Life on Earth makes use of only a limited number of diastereomers of all those that are possible. Moreover, biotic processes display an enantiomeric excess; e.g., left-handed amino acids and right-handed sugars almost exclusively predominate in living systems. Carbon atoms bearing four different substituents are said to be chiral centers. If a molecule has n chiral centers it will, in most cases, have 2n stereoisomers. There will, for example, be 256 stereoisomers of a compound with eight chiral centers. Each will have exactly the same chemical formula and pattern of connectivity among its atoms (A is connected to B is connected to C and D, and so on). Only the arrangements of those atoms in space will differ, and there will be 256 variations. Life functions by using only a small subset of all possible stereoisomers. ![]() FIGURE 7.1.1 An illustration of stereoisomerism. In these depictions of tetrahedral carbon atoms, bonds represented by straight lines lie in the plane of the paper. Those represented by wedges project in front of the paper (the filled wedges) or to the rear (the broken wedges). W, X, Y, and Z represent different chemical groups, anything from a single atom (an H, for example) to a complex chemical substituent with many atoms in addition to the one that is bonded directly to the carbon atom. In structure 7, all four groups are different. The mirror images, 7a and 7b, cannot be rotated so that the structures are superimposable. In structure 8, by contrast, two of the groups are identical. If structure 8b is rotated 180° about its vertical axis, it can be superimposed on 8a (i.e., it is seen to be identical to 8a). Mirror images of tetrahedra will be nonsuperimposable only when all four vertices are different. SOURCE: Reprinted from National Research Council, 2007, Exploring Organic Environments in the Solar System, The National Academies Press, Washington, D.C., p. 13. |
Enantiomeric excess can be detected in a number of ways. Direct observation of optical activity, that is, determination of the specific rotation [α]d of a compound, is cumbersome. Biochemical detection is also possible, although methodologies are generally specific to individual compounds or compound types. Indirect measurement through a chromatographic procedure, for example, gas chromatography or gas chromatography-mass spectrometry on a chiral stationary phase, has wide applicability and is very sensitive.
Chirality is often viewed as the unique province of biological systems. For this reason, researchers were surprised to find that several of the amino acids found in the Murchison meteorite were slightly enantiomerically
TABLE 7.1 Enantiomeric Enrichments for Amino Acids in the Murchison and Murray Meteorites
|
|
Enantiomeric Enrichment (%) |
|
Compound |
On Eartha |
Murchison |
Murray |
2-Amino-2,3-dimethyl-pentanoic acid |
|||
2S,3S/2R,3R |
Unknown |
7.6 |
1.0 |
2S,3S/2R,3S |
Unknown |
9.2 |
2.2 |
α-Methylnorleucine |
Unknown |
4.4 |
1.8 |
α-Methylnorvaline |
Unknown |
2.8 |
1.4 |
α-Methylvaline |
Unknown |
2.8 |
1.0 |
Isovaline |
Rare |
8.4 |
6.0 |
Norvaline |
Rare |
0.4 |
0.8 |
α-amino-n-butyric acid |
Common |
0.4 |
−0.4 |
Valine |
Ubiquitous |
2.2 |
−0.4 |
Alanine |
Ubiquitous |
1.2 |
0.4 |
aNatural abundance of the amino acid in Earth’s biosphere. SOURCE: Data from Pizzarello, S., and Cronin, J.R. 2000. Non-racemic amino acids in the Murray and Murchison meteorites. Geochim. Cosmochim. Acta 64:329-338. |
enriched (Table 7.1). Especially notable were enantiomeric enrichments of 7 to 9 percent in three specific amino acids not known in forms of life currently on Earth, thus making contamination from terrestrial sources unlikely.
If the amino acids in Murchison were not generated by living processes, it is possible that enantiomeric enrichment can be achieved by processes that are independent of life. Several such processes are known in the laboratory, some of which involve crystallization, or autocatalysis, and/or some multiple steps requiring human intervention. It is conceivable that some of these processes involve selective degradation of one of the two enantiomers. This weighs against the usual thinking that enantiomeric enrichment is a unique signature of life.
However, the data on enantiomeric excess are also consistent with a biotic origin, The larger asteroids may have stayed clement inside for several hundreds of millions of years, raising the possibility that life, or some borderline form, emerged there. This would be the case if the Murchison meteorite originated in an asteroid (or a comet) that supported life based on α-methyl amino acids that preferred one enantiomer to the other. While few would argue so, this result is consistent with life having emerged in the asteroid belt.
7.2
THERMODYNAMIC RELATION OF METABOLIC INTERMEDIATES AS A BIOSIGNATURE
For a metabolic sequence to convert substrates into products, the system in which they occur must not be at equilibrium. When they are in equilibrium, the overall reaction must be coupled to another reaction where substrates and products are at disequilibrium. This is frequently phrased as a requirement that a metabolic pathway be energetically “downhill,” or coupled to an energy source.
Many terran metabolic multistep pathways are run close to equilibrium for internal steps (Figure 7.1), with the first step being energetically downhill and a site of regulation. Required, however, is that the last step be energetically downhill, thereby pulling the reaction to completion.1 This feature may be universal in metabolic pathways simply because it exploits most economically a surrounding chemical disequilibrium. Having large drops in free energy at every step in a pathway is wasteful.
This characteristic energetic relationship between a set of compounds that are intermediates in an evolved metabolism may be a universal biosignature. If an inventory of the small molecules in a suspected living system (on Titan, for example) reveals this characteristic energetic relationship, this may be evidence of Darwinian evolution acting to create an optimal metabolism.
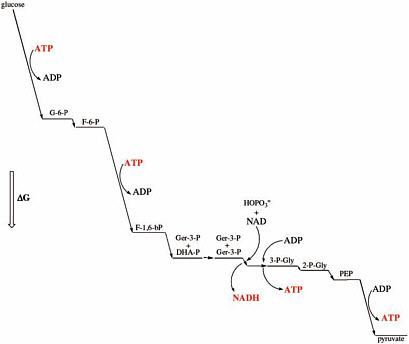
FIGURE 7.1 Physiological free energy diagram for the glycolysis reaction for one of the two glyceraldehyde-3-phosphate generated in glycolysis. G, glucose; G-6-P, glucose-6-phosphate; F-6-P, fructose-6-phosphate; F-1,6-bP, fructose-1,6-bisphosphate; Ger-3-P, glyceraldehyde-3-phosphate; DHA-P, dihydroxyacetone-phosphate; 3-P-Gly, 3-phosphoglycerate; 2-P-Gly, 2-phosphoglycerate; PEP, phosphoenolpyruvate. Vertical height is proportional to the change in free energy (ΔG).
7.3
REFERENCE
1. Voet, D., and Voet, J. 2004. Biochemistry. Wiley and Sons, Hoboken, N.J.