6
Observations and Science Potentially Enabled by the Vision for Space Exploration
The Vision for Space Exploration (VSE) and the returns to the Moon by robotic and human explorers will provide the opportunity to make use of the Moon’s location and the unique perspective that this location provides to carry out research in several fields of science. In this chapter the committee considers the potential benefits for various disciplines—astrophysics, gravitational physics, cosmic-ray physics, astrobiology, earth sciences, heliophysics, and magnetospheric and ionospheric physics—from of emplacing experimental apparatus on the Moon.
This committee was not constituted to recommend priorities for research in these diverse disciplines. The committee believes that the appropriate standing committees of the Space Studies Board are better able to judge the scientific priorities of research within their disciplines in the context of such research conducted from other locations in space, as well as the Moon.
ASTRONOMY AND ASTROPHYSICS
The lunar surface has historically been seen as a possible site for telescopes operating panchromatically and at the highest resolution, without an intervening absorbing and distorting atmosphere. In the early years of space astronomy, the stability of the lunar surface and large reaction mass were considered highly advantageous for telescope pointing and tracking, and the long diurnal cycle was considered advantageous, at least relative to low-Earth orbit (LEO), for thermal equilibration of large optical instruments. In addition, hypothetical future human visits to the Moon ensured some level of accessibility for service, maintenance, and upgrades.
The full costs of construction, deployment, and operation of telescopes on the lunar surface can reasonably be assumed to be larger than the costs for comparable equipment operating in free space, however. This cost difference arises, at minimum, from added propulsion requirements and the mitigation of added risks from landings. The issue then becomes a question of what features the lunar surface uniquely offers astronomical telescopes that free space does not.
The lunar surface offers, at least in the early stages of lunar development, extraordinarily radio-quiet sites on the lunar farside that could enable a highly sensitive low-frequency radio telescope.1 Such a telescope would be a
powerful tool in investigating the “dark ages” of the universe, before the reionization era, in which highly redshifted 21 cm (1420 MHz) emission from neutral hydrogen would reveal the earliest structures in the universe before the first phase of nuclear enrichment. At redshifts on the order of one hundred, this strong emission line approaches the ~15 MHz plasma frequency of Earth’s ionosphere, below which the opacity is very high and observations from the surface of Earth are not possible. For redshifts below this, the science thrust is for terrestrial efforts (e.g., Low Frequency Array, Square Kilometer Array), although such implementations are handicapped on Earth compared with the lunar farside, because of the severe human-produced and ionospheric radio-frequency noise. These low frequencies, unobservable from Earth, have special relevance also for heliophysical research on solar bursts and particle acceleration processes within them. A low-frequency radio interferometer with simple dipole elements spread out on a kilometer scale of the lunar surface thus has synergistic value to both priority astrophysical and heliophysical research. As a result of this synergy, as well as the usefulness of such low frequencies to understanding particle acceleration mechanisms in active galaxies, a modest nearside array has substantial scientific value both for these bright astronomical sources and also for allowing the refinement of technology and understanding of environmental issues.
An innovative concept recently proposed would have a complete antenna line electrodeposited on a long strip of polyamide film. In this form, a lightweight array could be simply unrolled onto the lunar surface in one of the first lunar return trips (see Figure 6.1). Unlike efforts in the optical, infrared (IR), or ultraviolet (UV) domains,
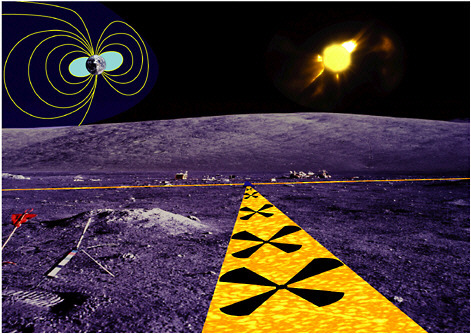
FIGURE 6.1 An artist’s conception of the Radio Observatory for Lunar Sortie Science farside Mylar dipole radio telescope (shown in yellow); an active Sun is shown on the upper right, and Earth’s magnetosphere is shown at the upper left. SOURCE: Courtesy of J. Lazio, Naval Research Laboratory; R. MacDowall, NASA/Goddard Space Flight Center; J. Burns, University of Colorado; D. Jones, Jet Propulsion Laboratory; K. Weiler, Naval Research Laboratory; and J. Kasper, Massachusetts Institute of Technology.
such a telescope would not be affected by dust deposition. As a result of the high astronomical priority of this work and the uniquely enabling character of the radio-quiet farside lunar surface, such efforts deserve cultivation.
With this in mind, near-term studies should be started to improve the understanding of the requirements and possible limitations of such a low-frequency radio interferometer effort, perhaps defining near-term site survey experiments that would help clarify the potential. What are the optimal sites for such an installation? There is also need to extend the original 40-year-old work that identified the “quiet zone of the Moon” and which is the only source of data on this subject to this day. What are the temporal characteristics of the frequency environment? To the extent that wide access to the lunar surface is not provided in the current VSE architecture, how close can such a facility be to the planned polar outpost and still have optimal performance? Given that farside human landings are not currently planned, how would such a facility be deployed and operated? Can a credible design for a major installation be developed that does not assume pre-existing infrastructure (communications, power, and so on)? With the recent predictions that sunlit/shaded edges of the Moon will develop substantial electrical potential gradients, are electrostatic discharges a possible natural noise source?
Currently, conventional single-aperture, single-spacecraft telescopes in free space that do not require precision constellation management now use proven pointing and tracking technology. The platform of the Moon is by no means as enabling for astronomy as it was once thought to be. Lunar gravity, while small compared with that on Earth, nevertheless requires that telescopes on the Moon be more massive than those based in free space in order to achieve a requisite stiffness that can preserve optical alignment as the telescope moves across the sky. However, a large telescope can be expected to have its surface shape maintained by active optics, and the issues of maintaining the surface by inertial reaction to the structure and the problem of free vibrations of that structure become larger as the telescope becomes larger. In the range of telescopes as large as the James Webb Space Telescope (JWST), such considerations seem to favor free-space locations. For larger apertures these issues will need to be re-examined. The frigid conditions in lunar polar craters might serve the needs of future thermal infrared telescopes, but passive cooling strategies now being designed into JWST for Earth-Sun Lagrange point L2 provide such low temperatures at modest fractional cost.
For ultraviolet astronomy and for astronomy involving the precise control of scattered background emission (e.g., planet detection), tolerance for dust contamination is very small. For thermal infrared astronomy, the observational background (which determines the sensitivity) is proportional to the absorbtivity (and re-radiation), not the reflectivity, so small dust deposits on the optics can seriously compromise the performance. As a result, the lunar surface appears to be minimally suitable for large UV/optical/IR telescopes.
Accessibility by humans is not clearly a unique advantage offered by the lunar surface. There is a large base of experience in deploying, maintaining, and servicing large telescopes in free space from the Hubble Space Telescope, as well as substantial expertise in in-space construction of large facilities from the International Space Station experience. The lunar-return implementation architecture for the Vision for Space Exploration, now in development, does not provide explicitly for in-space opportunities. Nevertheless, it appears likely that telescopes in free space, whether in LEO or at the dynamically convenient and thermally highly optimal Earth-Sun Lagrange points, may eventually be serviceable by humans and robots using augmentations to this architecture. In the case of remote Earth-Sun Lagrange points, such access could most conveniently be ensured after returning these telescopes by known pathways that require only small changes in spacecraft velocity to get to closer sites, such as the Earth-Moon Lagrange points. In this context, however, the lunar surface may well play a key role in operational support for such relocation efforts.2
The lunar surface gravity and solid surface do offer potential advantages for particular lunar observatory architectures and deserve further consideration by way of trade-off studies against functionally comparable free-space facilities. Several concepts may be viable, at least in the long term. A large liquid mirror telescope on the lunar surface near a pole that could offer extremely deep observations into very limited regions of the sky near the local
2 |
The development of a heavy-lift (Ares 5) launcher could offer major advantages to astronomy, providing the ability to lift large telescopes, fully assembled, into free space. In these respects, the astronomy community is particularly excited about the space transportation capabilities that will arise as the results of the lunar exploration architecture. See, for example, the proceedings of the Astrophysics from the Moon conference, Space Telescope Science Institute, 2007, at www.stsci.edu/institute/conference/moon, and the Lunar Exploration Science Workshop, Tempe, Arizona, February 27-March 2, 2007, at https://www.infonetic.com/tis/lea/, accessed May 29, 2007. |
zenith has been proposed. While highly innovative, the concept is technologically challenging, requiring a liquid with very low freezing temperature (<100 K) that can be flash coated, as well as a large precision-rotating tray for it. The very small field of view thus far proposed in the optical designs is, however, a significant handicap in meeting current National Research Council decadal survey science priorities.3 The surface also offers, as a solid optical bench, one strategy for deploying a multi-telescope, optically coupled interferometer that would offer high spatial resolution for astronomical sources. Such a design follows the pattern of terrestrial interferometers. However, a free-flying array in space could acquire data from a wider range of spatial frequencies than the range accessible to an interferometer fixed on the Moon. While lunar ultraviolet, optical, or infrared interferometers might be achievable with technology similar to that being used on Earth, the telescope emplacement, precise linkage, and sophisticated fringe tracking are not easy, and technology developments providing capability in free space would offer more potential. To the extent that such telescopes might someday be considered to be truly viable for priority astronomy, a careful assessment of dust contamination, both from natural processes and from human operations on the surface, will be necessary.
Finally, can the very substantial computing requirements of a future array for cosmological studies be credibly powered at a remote lunar site that is unlikely to see continuous solar illumination, especially in view of the fact that the quietest radio-frequency conditions will be at night? In addition, serious consideration needs to be given to the preservation of low radio noise on the farside. Are there strategies that can offer high-performance lunar global communication (e.g., laser communications) that will minimally compromise the scientific resource on the Moon as the Moon is developed?
Recent creative suggestions for astronomical instrumentation in lunar orbit deserve special mention. With no atmosphere to blur the horizon, sensor payloads in lunar orbit can use the edge of the Moon as an occulting knife edge for the precise localization of high-energy photons. With a detector spacecraft in lunar orbit, beam cutoff timing provides a source position in one direction. With apsidal plane motion of the orbit, many parts of the sky can be examined in different position angles, allowing a small spacecraft in lunar orbit with only modest pointing control to achieve very high angular resolutions for astronomical sources. The edge of the Moon has also been suggested as a convenient occultation strip to block out the Sun while doing deep infrared imaging in its vicinity in order to measure zodiacal dust at <1 astronomical unit (AU). Such instrument packages may be quite small and might find early use as attached payloads on future lunar surveillance orbiters, as well as on Crew Exploration Vehicle service modules that are parked in lunar orbit.
Other novel ideas for astronomical telescopes have been proposed in which the lunar regolith itself can be used as a high-energy particle and gravitational wave detector. While the lunar regolith is potentially enabling in this regard, the experiment would require substantial civil engineering in the form of excavation, drilling, and the movement of large volumes of regolith.
In the near term, opportunities for very small, “suitcase astronomy” (<100 kg) payloads, comanifested with equipment on an LSAM, might offer some astronomical value. A particularly potent example that does not even require such external supply equipment is evident in laser ranging reflectors. Such reflectors, as deployed on the Moon in the Apollo program, provided important data on the lunar orbit and (see Box 6.1) are now challenging understanding of basic physics. Other comanifested instruments might use power and communication from the Lunar Surface Access Module or outpost and be self-sufficient with regard to pointing and tracking. Although the committee is not aware of any such instrumentation that would address decadal survey priority astronomy (because the collecting areas are necessarily very small), a “free ride” to a lunar platform with a very modest, competitively selected instrument might offer science value. The opportunity presented by such small instruments as attached lunar payloads will have to be carefully evaluated with respect both to conventional implementation in free space (e.g., NASA’s Small Explorer (SMEX)-Lite and University Explorer missions) and to attachment to small missions at the Moon-Earth Lagrange point L1, or in lunar orbit.4
BOX 6.1 Lunar Laser Ranging: An Example of an Enabled Experiment Lunar laser ranging (LLR) measures the round-trip travel time of short laser pulses that are reflected back to Earth from corner-cube (retroreflector) arrays on the Moon. The range data are used to perform a general phenomenological check on our understanding of gravity—the weakest of the fundamental forces of nature. They also enable the refinement of the constants in the parameterized post-Newtonian formulation of general relativity and the testing of other theories of gravitation. LLR has provided the most precise limits to date on the following properties of gravity:
The first placement of retroreflectors on the lunar surface was by the Apollo astronauts in 1969. Recently, the Apache Point Observatory Lunar Laser-ranging Operation (APOLLO) began achieving 1 mm precision on lunar range. Deployment of next-generation reflectors would allow range precision to approach the 0.1 mm level, a better than two orders-of-magnitude improvement over the data used to determine the limits on the gravitational properties listed above. Placement of a single new array would be helpful, although by itself incapable of adequately constraining lunar rotational and tidal motions. Placement of three total new arrays widely distributed near the limb would optimally benefit high-precision ranging. The distribution of such reflectors/transponders may naturally and economically be associated with the implementation of a geophysical network. In addition to probing the predictions of gravitational physics, the rich LLR data set can be used for other scientific purposes. For example, by monitoring the physical reactions to known torques on the Moon, the properties of the lunar interior, such as the presence of a liquid core and the interaction between this core and the surrounding mantle, may be discerned. The LLR data series can also be used to study the orientation of Earth in space, which connects to climate monitoring, and geophysics. Solar system navigation also benefits from the LLR data series. SOURCE: Adapted from T.W. Murphy, University of California, San Diego, “Lunar Reflectors for Tests of Relativity,” white paper for the committee, 2006. |
There are near-term scientific investigations that would clarify some of the issues noted above. Of primary interest are studies of lunar dust resulting in a clear understanding of the threat that dust produced by exploration activity and natural processes poses to optical and mechanical systems and how dust enhances sky backgrounds, as well as strategies for mitigating this threat. Also needed is long-term characterization of the natural seismic environment, to be complemented by predictions of the induced seismic environment caused by future human lunar operations. A careful assessment of radio noise on the lunar farside, which may see contributions from local electrostatic discharges, is also of importance.
Thus, there are synergistic opportunities for combining site testing for astronomy with studies related to Earth observations and research on the lunar dust and plasma environment to yield data of interest to several scientific disciplines.
ASTROBIOLOGY
Astrobiology concerns the origin and development of life, the existence of life elsewhere, and the future of life on Earth and in the universe. There is great overlap between the aims of astrobiology and the disciplines
of astronomical, planetary, geological, and biological sciences; the significance of astrobiology comes from the integration of these areas. The origin and evolution of planets, the development of planetary crusts, and the impact and radiation environment that planetary bodies experience are critical boundary conditions for the habitability of Earth and other planets. A report by the NASA Astrobiology Institute5 outlines the relevance of the Moon to astrobiology, and many of the goals and objectives of the Astrobiology Roadmap6 are addressed by investigations recommended throughout this report.
The nature of the Moon and its accessibility provide a unique opportunity to make significant advances in several aspects of astrobiology. The importance of understanding the evolution of the lunar crust and other aspects of the Moon as a planetary end member is well recognized. Two specific areas have been called out for particular attention in the lunar context: (1) the early and recent impact flux and (2) the history of the lunar (and hence terrestrial) radiation environment.
The Moon provides a unique environment with which to study the early and late impact flux at 1 AU. The importance and influence of large, basin-sized impacts on the early evolution of life remain unresolved, and whether Earth experienced a late spike in basin-scale impacts (the terminal cataclysm) or a rate more smoothly declining is a critical boundary condition in this debate. Thus, the finding in this report that a South Pole-Aitken Basin sample return can make a vital contribution to the existence of a cataclysm would result in a major contribution to a high-priority astrobiological goal. However, the recent impact flux, well preserved and exposed on the Moon, is relevant to the development of life over the Phanerozoic, as impacts are a strong candidate for the cause of several major extinctions. Absolute age dating of stratigraphically important recent craters and a statistical sample of dated smaller craters would definitely determine the recent flux constraining the influence of impact on the evolution of life (extinction and evolutionary radiation).
The second identified priority for lunar astrobiological investigations is that of understanding the past energetic particle and plasma environment of the terrestrial planets. The particle flux is a critical unknown in understanding the ancient habitability of Earth. The early Sun was expected to have had a more intense solar wind and solar flare flux at early times, while the solar system has been repeatedly exposed to supernova and gamma-ray burst events and experiences a variable cosmic-ray flux as the Sun travels through the interstellar medium. Ancient lunar regolith recorded this environment in the form of trapped gases, fission products, and tracks. Sampling and analysis of ancient soil trapped between datable lava flows is the only known way to study the special circumstances at that critical time. While such sampling may be ambitious for an early robotic program, candidate sites can be identified and thoroughly characterized using high-resolution imaging and remote sensing for later detailed study.
Additional areas recently identified to be of high scientific interest to astrobiology include (1) development of understanding of organic chemistry in the lunar cold traps, (2) characterization of extrasolar planetary bodies with large lunar telescopes, and (3) search for possible components of ancient Earth in lunar regolith. The latter two are linked to the long-term potential of using the Moon for science as VSE infrastructure and facilities are emplaced.
Evaluating and sampling materials from the unusual environment at the lunar poles have been identified as a lunar science goal and are discussed in Chapters 3 through 5 of this report. As a potential repository of solar system volatiles and organics, polar material feeds directly into astrobiology concerns. The astrobiological significance of polar samples places a greater emphasis on cryogenic sample return, or alternatively on an appropriately equipped laboratory facility at a polar outpost supported by human fieldwork.
In identifying and characterizing extrasolar planets, there is congruence of the objectives of astronomy and astrophysics with those of astrobiology-related observational goals, namely, to maximize the effective aperture of telescopes. In the near term, tasks related to understanding the lunar environment (dust mobility, seismic stability, and so on) that enable such facilities to be evaluated and planned apply to astrobiology concerns as well as to astronomy and astrophysics. Earth-shine observations have been used to observe Earth’s spectrum near full phase,
but they fail to provide phase information, nor do they allow polarimetric studies such as those needed to identify liquid water. In preparation for the study of extrasolar planets, there could be particular value in making synoptic observations of Earth. For example, the vantage from the Moon (or a similar orbit) could allow the monitoring of specific properties of Earth as it would appear as a single point object, going through phases. Such data might identify readily measurable properties that are diagnostic of our habitable world, such as looking for possible evidence of oceans from the polarization of Sun glint and of vegetation from any cyclical spatial variation of the strong chlorophyll absorption edge in the visible to near-infrared spectrum.
We know that small amounts of material are redistributed from one body to another by impacts (e.g., meteorites), and the Moon retains a record that extends over 4 billion years (perhaps earlier if there was no terminal cataclysm). On the Moon, however, impacts pulverize or melt most impactor material while repeatedly mixing the regolith and megaregolith. Very small amounts survive as mineral grains or rock fragments. Nevertheless, some materials, such as zircons, are particularly refractory and may be preserved in lunar regolith. Nonlunar zircons would be geochemically distinctive and, if found, they would provide information about timing, composition, and conditions on their parent body, possibly including the habitability of early Earth. Existing lunar samples might be used to evaluate whether such materials exist in a detectable amount and, if promising, methods could be developed for prospecting likely environments on the Moon and processing large amounts of lunar material to search for unusual components foreign to the Moon that could be returned to Earth for advanced forms of analysis.
The Moon may also constitute a testbed for astrobiological studies. The isolation of the Moon from Earth can be biologically quite complete, which could make the Moon an appropriate place to study biota in a fully sterile and sterilizing environment. This could include developing a lunar facility for assessing the potential biological impact of martian materials to Earth’s environment. The exceedingly low organic levels in lunar regolith also may allow the Moon to be a useful testbed for very sensitive life-detection technologies.
EARTH SCIENCE FROM OR NEAR THE MOON
Remote Sensing of Earth From or Near the Moon
Earth observations from the Moon offer the another opportunity to acquire unique data to look at Earth in a bulk thermodynamic sense, particularly as an open system exchanging radiative energy with the Sun and space, in a way never done before—“Earth as a whole planet” (as illustrated in Figure 6.2). This is a fundamental scientific goal with very appealing prospects for climate and Earth sciences in general. Climate research requires stable,
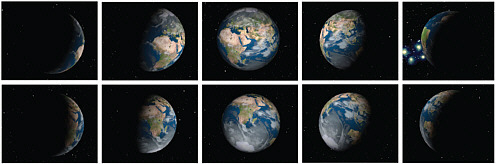
FIGURE 6.2 Simulated images of various phases of Earth as seen from the Moon over a period of days in both summer (top) and winter (bottom). SOURCE: Graphics created by Starry Night® and are used with permission from Imaginova Corporation. Starry Night is a registered trademark of Imaginova Corporation.
accurate, long-term observations made with adequate spatial and temporal resolution in a synoptic context. From lunar vantage points, it would be possible to sample the outgoing energy from virtually an entire hemisphere of Earth at once with high temporal and spatial resolution. At present this is only partially possible by combining data from LEO and geostationary orbit (GEO) satellites into an asynoptic composite of hundreds of thousands of pixels—rather like assembling an enormous jigsaw puzzle. Because of the integral view of the planet’s hemispheres continuously in the infrared and periodically at visible wavelengths, the observations will simultaneously overlap the observations of every LEO and GEO satellite in existence, making possible a unique synergy with great potential benefits for Earth sciences. One of the major potential benefits is the enabling of calibration sharing with all satellites, thus allowing the integration of all Earth-observing satellites in a single, integrated Earth-observing system that would provide self-consistent enriched data sets for Earth sciences. Such synergism would undoubtedly represent a major advance in Earth sciences and a greatly enhanced return for the nation’s investment in space and in particular for its investment in the exploration of the Moon.
Spectral observations from the Moon would allow for the first time continuous hemispheric synoptic retrievals of fundamental climate parameters, as well as data on cloud cover, aerosols, water vapor, and other atmospheric constituents, thus enabling unique observations of the diurnal cycle and its effects on the atmosphere. Note that observations in the IR are possible from all lunar orbital positions, while observations at visible wavelengths will be somewhat restricted.
Examples of possible instruments for observing Earth from the Moon are as follows:
-
Telescopes as a front end for spectroscopes to provide high spatial resolution imaging,
-
High-spectral-resolution spectrometers and/or interferometers covering the ultraviolet-visible-infrared spectrum, and
-
Reference radiometers.
The lunar view is nicely balanced in that it shows Earth rotating as well as going through its day-night phase alternation every 27 Earth days. Thus, both visual near-infrared observations that show solar reflected light and mid-IR observations that show Earth temperature could be obtained and would give a complete view to reveal changes such as El Niño–La Niña alternations and longer-term changes, and to reveal changes in the albedo of clouds with time.
Observations of Earth at relatively fine scale are possible with existing instrumentation. For example, a modest 2048 × 2048 pixel coverage of Earth has a pixel width of about 6.2 km. Yet the angular resolution required for this is possible with a 4 cm aperture at visible wavelengths. Even a resolution 10 times coarser would be adequate to put ~75 pixels across the United States and to watch weather patterns moving across Earth. Such observations would usefully allow cross calibration of the various geosynchronous orbiting weather satellite observations at 0.1 percent precision and so permit issues of the phase functions for observations to be resolved without recourse to theory.
In Situ Observations of the Variable Sun
Lunar exploration will provide opportunities to make use of the Moon itself to help develop a unified understanding of the radiative variability of the Sun (e.g., the constancy of the “solar constant”) on timescales of centuries. Drilling into the Moon’s regolith, if extended to a depth of 10 meters, will enable a measurement of the borehole temperature profile. The intent of the borehole heat flux experiment is to derive the history of the solar constant from the present-day temperature profile in the borehole and to gain knowledge of the thermal diffusivity of the regolith. The physics here involves the analysis of the transport of heat from the lunar surface down into the regolith. The effect of the constant heat flow from the lunar interior is removed by looking at deviations from the linear profile. This experiment was already attempted by Apollo 15 and Apollo 17, which succeeded in measuring the diffusivity but could only measure temperature to a depth of 2.7 m. Interpretation of a lunar borehole thermal profile is straightforward, providing a measure of variations in the solar constant extending back to the time of Galileo’s original 1610 mapping of sunspot areas, across the Maunder Minimum of sunspot numbers and
the “Little Ice Age.” Such an experiment would resolve the conflict among more indirect measures of the history of the solar constant, some indicating a significant brightening of the Sun since Galileo’s time and some claiming no brightening.
The information about the variation of the solar constant, derivable from the Moon’s regolith temperature profile, has important implications for the interpretation of Earth’s currently observed global warming and is a prerequisite to any estimate of humanity’s contribution to global warming.
HELIOPHYSICS OBSERVATIONS FROM OR NEAR THE MOON
Imaging of Radio Emissions from Solar Coronal Mass Ejections and Solar Flares
Radio observations of solar eruptions have played an important role in understanding the Sun, but the terrestrial ionosphere blocks all radio frequencies below 10 MHz to 20 MHz, which cover virtually all radio emissions originating above 1 to 2 solar radii from the Sun’s surface.
Multi-site observations of low-frequency radio signals from the Moon, where there is no blocking ionosphere, would allow imaging of the sites of particle acceleration in the extended solar corona. In this region, the primary radio sources are fast (2 keV to 20 keV) electrons from solar flares and suprathermal electrons (~100 eV) accelerated by shocks. The associated radio emissions are called Type III bursts and Type II bursts, respectively. Both sources produce a plasma instability, which leads to amplification of electrostatic waves, some of which are then converted to electromagnetic (radio) waves. The process takes place at the characteristic frequency of the plasma—the electron plasma frequency—thus, the frequency of the radio emission indicates directly the electron density of the source, and imaging the radio source would map the extent of the acceleration region.
To make such images at low frequencies would require a synthetic aperture that is large compared with the wavelengths of interest. An angular resolution of 1 degree at 1 MHz requires a minimum diameter of 15 km. The Moon offers a large, stable surface on which to build a large, capable low-frequency radio array for the purpose of imaging solar sources at wavelengths that cannot be observed from the ground, an array that is well beyond the current state of the art for antennas in space. Figure 6.1 illustrates an antenna concept, operating at somewhat higher frequencies for radioastronomy purposes, being deployed on the Moon.
The design for the first (test) array would be based on the designs of Earth-based arrays (working at higher frequencies) currently in development. The deployment of the initial test elements could be done by astronauts or robotic deployers or both. Subsequent deployment of additional elements of the array would be carried out over time, permitting one to maximize lessons learned from implementing each phase.
The major components of the observatory are the antenna array and electric connections, the radio receivers, the central processing unit, the high-gain antenna unit, and the power unit. For a test array with 16 to 32 antennas, the expected total mass for a lunar observatory would be significantly less than 400 kg, and the power requirement would be less than 500 W.
Imaging of Earth’s Ionosphere and Magnetosphere
Were it visible to the naked eye, Earth’s magnetosphere would be the most spectacular object in the lunar sky. Subtending about 20 degrees at Full Earth and gradually expanding to envelop the entire lunar sky near New Earth, the magnetosphere would be an awe-inspiring phenomenon. Elements of the magnetosphere can, however, be imaged from the Moon through the use of modern instrumentation on timescales and at spatial resolutions of great interest to magnetospheric plasma physicists and specialists in space weather analysis and forecasting.
A unique advantage of the lunar perspective would be the ability to stare at the space neighborhood of Earth, providing global long-term images of the time development of extended magnetospheric phenomena such as the plasmasphere and ring current. The time period for significant evolution of these phenomena is a few hours, a time interval over which the perspective of an Earth-orbiting satellite changes significantly, while observations from the Moon do not vary appreciably in perspective.
Since the launch of the Imager for Magnetopause-to-Aurora Global Exploration (IMAGE) satellite in 2000, images of Earth’s magnetosphere and ionosphere have become powerful tools in the investigation of the global dynamics of geospace. While high-latitude phenomena, such as the aurora, cannot be imaged all of the time effectively from the Moon, many other important magnetospheric and ionospheric phenomena at lower latitudes can be imaged for sufficiently long times to be of great interest. The lunar perspective would provide unique information in the area of space weather diagnostics.
The basic tools for geospace imaging are ultraviolet radiation and energetic neutral atom (ENA) cameras. With UV, emissions from specific ion species can be used to image large regions of the magnetosphere and ionosphere and to study the dynamics of these regions as the ions interact with terrestrial electric and magnetic fields.
ENA imaging is possible because Earth’s hydrogen exosphere extends throughout large portions of the inner magnetosphere, as was demonstrated dramatically by the Apollo images obtained at the Lyman alpha wavelength. All ion populations in the magnetosphere undergo charge-exchange interactions with the exospheric atoms with the result that a few percent of the ion population is converted to energetic neutral atoms. Since these atoms are not affected by Earth’s magnetic field, they can be imaged just as photons are imaged. Images formed by energetic neutral atoms provide global views of magnetospheric ion dynamics sorted by energy, velocity, and species.
Ultraviolet imaging from the Moon can reveal the dynamics of the plasmasphere, which is the upward extension of the ionosphere. The plasmasphere consists principally (about 85 percent) of protons, which cannot be imaged, but the remaining fraction is mostly He+ ions, which can be imaged through resonantly scattered sunlight at a wavelength of 30.4 nanometers. Plasmasphere images are crucial space weather diagnostics, because during magnetic storms, giant plumes of plasma grow out of the plasmasphere as the plasmasphere itself shrinks. These plumes map along magnetic field lines into the ionosphere, producing enhancements of total electron content, which have been shown to seriously disrupt communications as well as Global Positioning System navigation signals. Examples of plasmasphere images taken from a low-latitude perspective are shown in Figure 6.3.
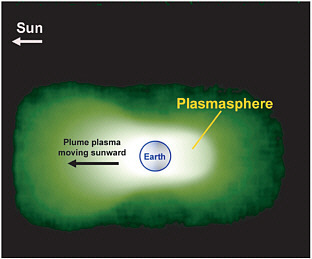
FIGURE 6.3 Simulated view of the He+ portion of Earth’s plasmasphere, as it would appear to a proposed second-generation Imager for Magnetopause-to-Aurora Global Exploration (IMAGE)-Extreme Ultraviolet instrument camera system placed on the lunar surface. SOURCE: Courtesy of Dennis Gallagher, NASA Marshall Space Flight Center.
Similarly, the low-latitude ionosphere can be imaged from the Moon by detecting the UV emissions of ionospheric ions. Emissions from O+ ions have been used to track the formation and evolution of density troughs associated with the ionospheric spread-F phenomena. The motions of the density troughs, as shown in Figure 6.4, can be used to measure the evolution of large-scale ionospheric electric fields.
Energetic neutral atom imaging was shown to be effective during the IMAGE mission over a very large energy range, from 10 eV to 500 keV. One of the most important imaging targets is the ring current, which builds up in timescales of hours during magnetic storms and is a key ingredient in the dynamics of Earth’s magnetosphere. An example of a ring current image taken from a low-latitude perspective is shown in Figure 6.5.
In summary, imaging from the Moon will provide a new perspective on magnetospheric and ionospheric dynamics because of the possibility of making observations from a vantage point providing a global view and for time spans substantially longer than the timescales of interest for these very dynamic phenomena.
The instrumentation for lunar-based geospace imaging would be based on the flight-proven IMAGE instruments with correspondingly larger apertures to allow for the approximately seven times larger distance. Multispectral UV and ENA imagers would require relatively modest resources, in the range of 50 kg to 100 kg and 30 W to 50 W each. Deployment could be done either robotically or by astronauts.
Finding 5: The Moon may provide a unique location for observation and study of Earth, near-Earth space, and the universe.
The Moon is a platform that can potentially be used to make observations of Earth (Earth science) and to collect data for heliophysics, astrophysics, and astrobiology. Locations on the Moon provide both advantages and disadvantages. There are substantial uncertainties in the benefits and the costs of using the Moon as an observation platform as compared with alternate locations in space. The present committee did not have the required span
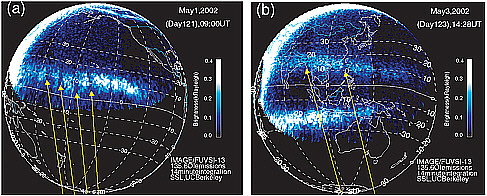
FIGURE 6.4 Oxygen airglow images for (a) May 1, 2002, and (b) May 3, 2002, showing nightside equatorial plasma bubbles whose drift motion can be used to determine ionospheric electric fields. (The arrows identify “bubbles” in the ionosphere, which are used to determine ambient plasma drift speeds in the second part of the figure, not shown here.) Data from Imager for Magnetopause-to-Aurora Global Exploration (IMAGE)-far-ultraviolet instrument. SOURCE: Courtesy of T.J. Immel, H.U. Frey, S.B. Mende, and E. Sagawa, Global observations of the zonal drift speed of equatorial ionospheric plasma bubbles, Ann. Geophys. 22:3099-3107, 2004.
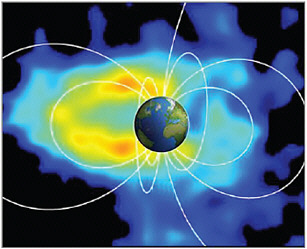
FIGURE 6.5 Energetic neutral atom (ENA) image at ~20 keV to 50 keV from an equatorial perspective. Data from Imager for Magnetopause-to-Aurora Global Exploration (IMAGE)-high-energy neutral-atom imager instrument. SOURCE: Courtesy of J. Burch, Southwest Research Institute, D. Mitchell, and P. Brandt with support from NASA under contract NAS5-96020.
and depth of expertise to perform a thorough evaluation of the many issues that need examination. A thorough study is required.
Recommendation 5: The committee recommends that NASA consult scientific experts to evaluate the suitability of the Moon as an observational site for studies of Earth, heliophysics, astronomy, astrophysics, and astrobiology. Such a study should refer to prior NRC decadal surveys and their established priorities.