SESSION 4
SOLAR PUMPING OF LASERS
Lloyd Chase
Lawrence Livermore National Laboratory
Livermore, California
Rapporteur's Report
The conversion of sunlight into laser light by direct solar pumping is of potential importance because broadband, diffuse, temporally constant, sunlight is thereby converted into laser light, which can be a source of narrowband, collimated, rapidly pulsed, radiation with the possibility of obtaining extremely high brightness and intensity. Nonlinear processes, such as harmonic generation, might be used to obtain broad wavelength coverage, including the ultraviolet wavelengths, where the solar flux is very weak. Solar pumping of lasers was first demonstrated in 1963, but most of the technologically significant effort has occurred during the past decade. There are presently few research efforts in this area, and the ultimate performance and potential applications of this technology are relatively unexplored. The three presentations in this session illustrated three different methods for concentrating the solar flux to facilitate pumping of various laser media. These methods are (1) imaging concentrators, (2) nonimaging concentrators, and (3) blackbody cavity pumping. A few important applications for solar pumped lasers were identified but there was not adequate information presented regarding technical and cost considerations so that the suitability of solar pumped lasers could be assessed.
The paper by Winston demonstrates the use of nonimaging concentrators to obtain solar light fluxes several times larger than those possible by direct imaging. This improvement is important because the size of the solar collector required to achieve laser threshold is reduced, and laser operation might be possible with some media with pump thresholds that are too high for practical operation with imaged concentrators. Dye lasers are a good example of such a medium. Several of the topics discussed in this paper were debated by the discussants, and they merit further investigation. One of these topics was the application of the second law of thermodynamics to predict the maximum brightness gain of solar pumped lasers. It was pointed out in the presentation by Lee that the lasing threshold for a favorable dye such as rhodamine 6G is about 10,000 solar constants, not very far below the maximum pumping intensity that can be achieved with the nonimaging concentrator, and the lasing threshold must be exceeded by a large factor to give high overall efficiency. The question of the performance achievable with solar pumped dye lasers will soon be addressed by experiments to be performed by Dr. Winston's group at the University of Chicago. A third topic of debate was the application of solar pumped dye lasers to laser isotope separation. Dr. Winston's cost estimates for this technology assume an overall dye laser efficiency of 5%. However, the implementation of laser isotope separation in its current form requires laser pulses with a fairly low duty cycle. This is a mode of operation in which solar pumped lasers are very inefficient, because the low duty cycle wastes much of the collected solar power. This question must be considered and, if necessary, its impact on the cost estimates should be included.
In the paper by Christiansen the novel idea of pumping lasers with the solar radiation trapped in a blackbody cavity is discussed and demonstrated. In principle, the recycling of the radiation trapped in the blackbody cavity might provide much more efficient utilization of the collected solar flux than direct solar pumping. This is illustrated by the relatively high efficiency predicted by Christiansen for a neodymium activated solid state laser. It remains to be demonstrated, however, that this advantage can be achieved in practical blackbody cavities. The loss of thermal energy from the blackbody must be not much larger than, and preferably smaller than, the laser output in order to achieve the predicted efficiencies. Such a demonstration should have a high priority in future research plans. A potential advantage of blackbody pumping is that the collected solar energy is stored in the blackbody during periods of interruption of the solar flux, during which laser operation might continue, perhaps with the infusion of substitute forms of heating of the blackbody during long interruptions.
The solar pumped gas laser discussed by Lee uses an imaging concentrator, which is adequate for pumping gases of molecules containing iodine to above their laser threshold. The utility of such a laser for space-based applications, such as communications and power transfer to space vehicles is being explored. This laser concept has the advantage of readily scaling up to large sizes for high output powers. The anticipated efficiency of this type of laser (about a tenth of a percent or so) is rather low for prospective terrestrial applications in competition with other technologies.
At this stage of their development, it might be said that solar pumped lasers are a possible solution in search of possible problems. While judging the merits of solar pumping of lasers for any application, it is, of course, important to keep in mind the competing technologies that employ either solar or nonsolar energy sources. For example, semiconductor diode laser pumped solid state lasers powered by solar pumped photoelectric cells may provide stiff competition in the future for direct solar pumping in prospective laser applications. At this time too little is known about the performance and cost of solar pumped lasers to make meaningful assessments of their suitability for applications or comparisons with other technologies. It is therefore important to shape research and development programs on the various solar pumping schemes with a view toward clearly establishing the limits to their performance. At the same time, efforts should be made to identify the promising applications of solar pumped lasers and to make credible assessments of their suitability for those applications. If progress is not made in parallel in these two areas, it may be difficult to come up with either the 'solution' or the 'problems' in this period of intense competition for funding.
SOLAR PUMPED LASERS: WORK IN PROGRESS AT THE UNIVERSITY OF CHICAGO
Roland Winston
Department of Physics
University of Chicago
INTRODUCTION
Of the variety of solar energy conversion schemes that have been explored, the conversion of solar flux to coherent laser radiation is a relative newcomer. Actually, demonstration of solar pumped lasing goes back to the 1960s[1]. However, the number of groups engaged in solar laser research is extremely small [2, 3]. Part of the reason for this is technological. Solar flux at sufficiently high concentrations to overcome threshold for the really important laser materials has simply not been available. This technological inhibition has recently been overcome through the application of nonimaging optics [4] through the demonstration of concentration levels of 84,000 ''suns'' at the University of Chicago in a refractive medium (sapphire) and of over 20,000 "suns" in air at the Solar Energy Research Institute (SERI) High Flux Facility [6]. One can therefore expect renewed interest and increased activity in solar lasers, this latest and least developed frontier of solar energy utilization.
THERMODYNAMIC OVERVIEW OF SOLAR LASERS
It is useful to view solar lasers in the context of other more familiar and more highly developed solar utilization technologies. The Second Law of Thermodynamics provides a useful unified framework for this purpose.
Thermal Cycle
In the most familiar of the solar utilization technologies, heat q1/T1 is delivered at temperature T1 while heat q2 and entropy q2/T2 is rejected at temperature T2. The difference q1-q2 is available for supplying work W. The Second Law (Increase of Entropy) implies that the efficiency will not exceed (1-T2/T1), the Carnot efficiency. A similar discussion could be constructed for the photovoltaic cell cycle.
Down-conversion Cycle
Photons of frequency ν1 and energy hν1 (h = Planck's constant) from a blackbody source at temperature T1 (the sun) are absorbed in material and re-emitted at a lower frequency ν2 and energy hν2. In this process (called fluorescence) the energy difference q = hν1-hν2 is delivered as heat at temperature T0, where T0 is essentially ambient temperature ≈ 300 K. Recalling that the brightness of blackbody radiation Planck spectrum can be written as

it follows that the entropy associated with hν, hν/T can be written as

where Bν is the specific intensity or "brightness." The Second Law (Increase of Entropy) implies the inequality:

where

Recalling that T0 (in our thermodynamic temperature units) is ≈ 0.025 eν while hν1-hν2 is, say, ≈ 1 eν, the exponential factor is truly large ≈ 1017! This then is what the solar laser does, increase brightness by a huge amount. Let us analyze how well the solar laser performs. Consider a primary that intercepts 100 kW of solar radiation and a laser that converts this to laser power with, say ≈ 10% efficiency. The brightness of the source, the sun, is Bν ≈ Bsun/Δν, where B is the brightness of the solar surface ≈ 2 × 107 W/M2.
The brightness of the laser is Bν = Pν/λ2Δν where Pν is the laser power, λ the wavelength and Δν the line width. Taking Pν = 10 kW, λ = 1µm and the ratio of linewidths (blackbody/laser) ≈ 106 (inverse ratio of coherence lengths), we obtain
Bν(laser)/Bν(sun) ≈ (104/2×107) (1/λ3) = 0.5 × 1015
so we see that the solar laser does pretty well at increasing brightness! While one might be left with an impression that the input numbers have been chosen to make the answer "come out right," the legitimate conclusion that can be drawn is that the solar laser operates near the Second Law limit of brightness gain.
Solar Laser Program at the University of Chicago
Solid-State Lasers
The solar laboratory at our university is situated on the roof of the high-energy physics building. A 1 m2 heliostat redirects solar radiation to a small primary reflector. (The heliostat is ordinary float glass covered with 3Z silver film.) The light from the primary is then further concentrated by a nonimaging concentrator. In our early work the primary reflector was a 40-cm diameter telescope mirror. The mirror was front-surface silvered and had a large enough focal ratio (f/2.5) so that it could be made spherical instead of parabolic, with little loss of concentration—and at considerably less expense. With an incident intensity of 800 W/m2, the mirror delivered about 100 W of power to the concentrator in the form of a spot approximately 1 cm in diameter.
Initially the concentrator was made of acrylic, which has an index of refraction of about 1.5. To keep the concentrator from melting, a spinning wheel with a hole in it was placed in front of the concentrator to chop the sunlight. The concentrator "compressed" the 1 cm spot from the mirror to a 1.27 mm diameter spot. One way of thinking about the experiment, then, is that all the light from the 40 cm diameter mirror was funneled' into a 1.27 mm diameter spot.
The light exiting the tip of the concentrator was then directed into one end of a laser crystal; the tip of the concentrator was optically coupled to the crystal. We worked with two types of crystals, Nd:Cr:YAG and Nd:Cr:GSGG. Both crystals were 30 mm long and 1.6 mm in diameter. Since the light exiting the tip of the concentrator forms a hemispherical front, the effective concentration area is increased from 1.27 to 1.6 mm, with a corresponding decrease in overall concentration. The coupled end of the laser rod was coated with a high-reflection (HR) film at 1.06 microns, the wavelength at which the neodymium atoms lase. The HR coated end served as the end mirror of the laser cavity. The other end of the crystal was antireflected (AR) coated at 1.06 microns. An external partially transmitting mirror completed the laser cavity. We successfully lased both types of crystals.
In the next stage of our research we sought to increase the efficiency of the Nd:Cr:GSGG system and to lase alexandrite, which has the advantage of being tunable. Two tactics were crucial to this work. First we switched from acrylic to sapphire, which has an index of refraction of 1.76. Since concentration is proportional to the square of the index of refraction, this boosts our concentration by a factor of (1.76/1.5) 2 ~ 1.4, resulting in a 1.13 mm diameter spot. Second, we decided to grind the outer diameter of the laser crystals to match the diameter of the concentrator tip and thereby avoid the loss in concentration due to oversizing of the laser rod.
Developing the required sapphire concentrators proved to be a significant challenge in itself. Sapphire is almost as hard as diamond, and is therefore difficult to work with. Nevertheless, in the past few years our learning curve for machining and polishing this material has rocketed almost exponentially. We owe our success in mastering this task to the excellent university machine shop and its extraordinarily skilled craftsmen.
Unfortunately, we discovered that grinding the laser rods to 1.13 mm in diameter was deleterious to the crystals: the crystals lased before grinding, but failed to work after. The grinding process apparently introduces stress into the crystals, which prevents lasing from occurring. Since no laser crystal supplier will supply crystals smaller than ~2 mm in diameter, we decided to upscale our system to accommodate 2 mm rods. This entailed going from the 40 cm diameter mirror to a 72 cm diameter mirror (which has a slightly smaller focal ratio, f/2.0, to keep the spot it produces in our laser "hut"). We also had to make the sapphire concentrators correspondingly larger. We are currently working on lasing Nd:Cr:GSGG and alexandrite. We are exploring the possibility of applying an HR coating to the alexandrite crystal as well. Putting the coating on the end of the Nd:Cr:GSGG crystal is acceptable because that crystal lases at 1.06 microns but absorbs light strongly at 400 and 600 nm, two bands far enough from 1.06 microns that they are not reflected. Alexandrite, however, lases over a band of frequencies centered around 750 nm, so that the HR coating could turn back the pumping radiation at 600 nm. We are looking for a coating that will be highly reflective at 750 nm, but highly transmissive below 700 nm.
Dye Lasers
In parallel with our work on solid-state lasers we are also seeking to demonstrate the feasibility of a solar pumped dye laser. A dye laser would have several advantages over its solid-state counterpart. A dye does not "break," and its circulation provides a natural cooling mechanism. Dyes are better at absorbing light from the solar spectrum, and they can be lased at lower wavelengths, around 600 nm. Such low wavelengths raise an interesting possibility: frequency doubling to ultraviolet radiation. Many industrial and photochemical processes employ UV radiation between 300 and 350 nm, which can be reached with a frequency-doubled dye laser. The doubling process, which is performed with a nonlinear crystal, would fall somewhat out of this range in the case of alexandrite, yielding an output around 375 nm.
As with the solid-state lasers, light from the 72 cm diameter primary mirror is directed into a nonimaging concentrator. In this case we have decided to work with acrylic again, because it is more easily machined. The acrylic "funnels" and "folds" the light onto a small slot 200 microns wide through which the dye can pass. The bottom of the slot will be coated with an HR mirror at 600 nm, forming the end mirror of the laser cavity, and an external mirror also coated for 600 nm will allow the laser beam to emerge.
As of this time we have designed, built, and coated the acrylic concentrator. A dye circulator is graciously on loan from one of the university research groups. We are now in the process of searching for a solvent for the dye (rhodamine 6-G). The solvent must have an index of refraction that matches that of acrylic (n ~ 1.5), it must not attack acrylic, and it should have a relatively low viscosity. Unfortunately, the first two requirements seem to always be at odds with each other, which is to say that solvents with a high index also attack acrylic. We may reach a compromise by
working with a slightly lower index solvent such as ethylene glycol (n = 1.43).
References
1. Young, C. G. 1965. Appl. Optics 5.993.
2. Yogev, A. et al. 1990. Optics Letters 15:36.
3. Arashi, H., et al. 1984. Jpn. J. Appl. Phys. 23:1051
4. Welford, W. T. 1989. High Collection Nonimaging Optics. Academic Press, New York and London.
5. Cooke, D., et al. 1990. Nature 346:802.
6. Lewandowski, A., et al. 1990. International Engineering Agency Symposium on Solar High Temperature Technologies, Davos, Switzerland, August 27–31.
BLACKBODY PUMPED LASERS
Walter H. Christiansen
University of Washington
Seattle, Washington
Summary
The principle of broadband optical pumping using flashlamps to achieve a working laser is well-known and has been put into practice with the ruby laser, Nd:YAG, and iodine lasers. While solar radiation can also be used to directly pump a lasant, only those systems with a broadband absorption spectrum may achieve satisfactory efficiencies, due to the very broad pumping spectrum. For example, the theoretical efficiencies of broadband directly pumped lasers, to date, are much lower than 2% under solar pumping conditions. Thus, solar pumping of lasers employing line or band absorption is inefficient because of poor utilization of the optical spectrum.
A different concept for a solar-powered laser is presented which utilizes an intermediate blackbody cavity to provide a uniform optical pumping environment for the lasant, typically CO or CO2 or possibly a solid state laser medium. In such a system, the entire solar flux could be used to pump the lasant. A schematic of the pumping arrangement is shown in Figure 1 where focused sunlight is used to heat a blackbody cavity. The cooled laser medium is positioned inside the cavity and pumped by the blackbody radiation. While a flowing medium is shown in Figure 1, the concept can also apply to a solid medium. The blackbody radiation cycle is shown in Figure 2. The blackbody radiation emitted by the walls (1) impinges on the laser medium. The medium absorbs a band of the spectrum, which results in the nonequilibrium spectrum (2). This radiation is then reabsorbed and thermalized by the walls and re-emitted in the original spectral distribution (3), and the process is repeated. In this way the pumping radiation is continuously replenished and all the energy of the source is used to pump the laser absorption bands. Only the nonlasing absorption bands of the medium and absorption by the laser tube walls limit this concept from providing high thermal efficiencies equal to the intrinsic laser efficiency of the laser medium.
The intermediate blackbody can be heated by solar radiation to a high temperature and its heat losses, other than to the laser medium and surrounding coolant tube, can be designed to be small. The laser medium and containment tube would be kept cool by circulating a coolant. A cavity temperature of 2000 to 3000 K seems possible using modern materials for the cavity and good concentrating optics. Thus, the radiation pumping spectrum is like a blackbody at the intermediate temperature. At temperatures of 2000 to 3000 K, the blackbody spectral intensity in the visible region is reduced markedly from that of sunlight. However, there is not so much reduction in the infrared region. This lower intensity might be thought to reduce the rate of pumping from that of direct pumping, except for the fact that the solid angle exposure of the tube is 4p, instead of being limited by the f number of the collector, and sideview pumping is easily achieved. It turns out that the reduction of the intensity of the pump radiation due to lower blackbody temperature is compensated somewhat by the increase in solid angle of the exposure of the laser tube in the cavity.
High power continuous wave (cw) blackbody pumped lasers with efficiencies on the order of 20% or more are feasible, provided transparent optical materials are available and lasing threshold is reached. The physical basis of this idea is reviewed. Small-scale experiments using a high temperature oven as the optical pump have been carried out with gas laser
mixtures. Detailed calculations showing a potential efficiency of 35% for blackbody pumped Nd:YAG systems will be discussed.
The feasibility of using intermediate blackbody cavity pumping has been demonstrated with a CO2 laser. The same system was used for testing N2O and isotopic mixtures of CO2. These experiments were conducted using cylindrical laser tubes with longitudinal flow, at 1500 K blackbody temperature. Finite optical depth of the pumping radiation and axial flow geometry limited the power of this system. Carbon monoxide has also been studied as a lasing species for a blackbody pumped system. Basic calculations performed have shown very high potential efficiencies and high absorbed power densities. Analytical and experimental studies were performed for mixtures of CO with Ar and N2. The details of the characteristics of CO as laser medium for a blackbody pumped system have been modeled and gains of 0.3/m have been predicted in some instances. However, lasing of CO has not yet been observed in the laboratory.
Researchers have observed cw lasing in Nd:YAG using broadband pumps such as flashlamps, hot tungsten filaments, and focused sunlight. The maximum measured slope efficiency ranges only from 1/2 to 2% using these optical pumps. The blackbody concept has been applied to this laser in an effort to improve its overall efficiency. The main cause of low efficiency can be remedied by using a blackbody cavity which rethermalizes the unused power. Laser efficiencies of up to 35% are predicted for a blackbody system whose pumping is provided by a thermal radiation source. If achieved in practice, this would represent over an order of magnitude improvement in efficiency for this laser. The threshold conditions for lasing will be discussed and scaling concepts for a high power system will be briefly mentioned.
To further progress on the blackbody concept, additional research on the following needs to be done:
-
Proof of principle experiments must be undertaken, that is, lasing while using a blackbody pumping source, especially the low threshold solid state media, that shows the promise of high efficiency.
-
Power scaling principles must be developed, based on experiments.
-
The use of modern high temperature materials for blackbody cavity design and heat insulation must be explored.
-
The properties of low absorption materials for the coolant laser tube and host laser material must be determined and developed by measuring the absorbed power fraction directly under blackbody conditions.
-
Low cost concentrating (i.e., low f) mirrors must be developed.
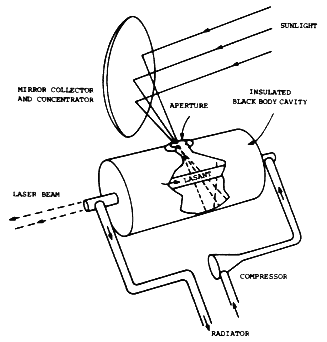
Figure 1 Solar-pumped laser via blackbody cavity.
Reprinted with Permission from: University of Washington, Department of Aeronautics & Astronautics
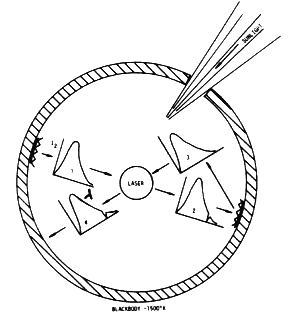
Figure 2 Thermalization of a nonequilibrium radiation field by the blackbody cavity.
Reprinted with Permission from: University of Washington, Department of Aeronautics & Astronautics
SOLAR PUMPED LASERS AND THEIR APPLICATIONS
Ja H. Lee
Nasa Langley Research Center
High Energy Science Branch
Hampton, Virginia
Solar Pumped Lasers
The first solar pumped laser was successfully demonstrated in 1963 immediately after the invention of the laser itself. Any laser material that can be photopumped could be considered for solar pumping in principle. However, until recently, solar pumped laser research and development attracted little attention. Since 1980, NASA has been pursuing high-power solar lasers as part of the space power-beaming program. Materials in liquid, solid, and gas phases have been evaluated against the requirements for solar pumping. Two basic characteristics of solar insolation, namely its diffuse irradiance (0.1 W/cm2) and 5800 K blackbody-like spectrum, impose rather stringent requirements for laser excitation. The maximum solar concentration achievable and limited utilization of the solar spectrum by the laser medium, which results in a large amount of waste heat removal, are disadvantages of artificial pump sources, such as flash lamps, arc lamps, and pump lasers.
However, meeting these requirements is not insurmountable as solar thermal energy technology has progressed today, and taking advantage of solar pumping of lasers (the solar energy is free, nonlocal, pollution-free, and abundant) is becoming increasingly attractive. Indeed, direct solar pumped lasers using existing solar furnaces have been reported from Japan (H. Arashi, 18 W, Nd:YAG, 1984) and Israel (M. Weksler et al., 100 W, Nd:Cr:GSGG, 1987; R.M.M. Benmair et al., 12 W, Er:Tm:Ho:YAG, 1989). The recent high-power accomplishment in the United States is the excitation of a 24 W continuous wave (cw) iodine photodissociation laser using an argon arc solar simulator at the NASA Langley Research Center. Increased interests in solar pumped lasers are indicated by numerous studies of solar laser concepts and system designs reported by researchers in Germany and the USSR, in addition to the countries mentioned above. (A brief review of these efforts will be given.)
Solar Pumped Laser Applications
The high-density photons of concentrated solar energy have been used for mainly electric power generation and thermal processing of materials by the DOE Solar Thermal Technologies Program. However, the photons can interact with materials through many other direct kinetic paths, and applications of the concentrated photons could be extended to processes requiring photolysis, photosynthesis, and photoexcitation.
The NASA solar pumped iodine laser program is based on photolysis of perfluoroalkyl iodides in the near-ultraviolet band (250–350 nm). Recently, the Langley team has evaluated the feasibility of using AM1 (terrestrial) solar spectrum for pumping the iodine laser and found favorable results with t-C4F9I in the threshold pump power, cavity design requirements, and laser output estimates. The references to be cited show that other laser systems using solid, liquid, and gas phases of laser media are also feasible with the existing solar concentrators of the Solar Energy Research Institute (SERI). once high-power lasers are produced at characteristic wavelengths of the system, there are many avenues, such as Raman shifting and harmonic generation, for modifying (tuning) the output to meet the photochemistry of target materials or processes.
The use of solar pumped lasers on Earth seems constrained by economics and sociopolitics. Therefore, prospective applications may be limited to
those that require utilization of quantum effects and coherency of the laser in order to generate extremely high-value products and services when conventional and inexpensive means are ineffective or impossible. For example, the destruction and removal efficiency (DRE) set by the government for toxic waste treatment requires conventional incinerator temperatures to be extremely high (~1600°C), and the cost of operating and maintaining such a facility is unbearably high. By using a solar incinerator with ultraviolet light for only a few percent of total insolation in the waste treatment, the temperature required for DRE is significantly reduced according to Graham et al. (1987). This report indicates that suitable photon energy (i.e., wavelength) exists for a given photochemistry, and use of a suitable laser will result in a significant increase in the treatment efficiency.
The readily developed high-power lasers by solar pumping are Nd:YAG (1.06 µm), CO2 (10.6 µm), and iodine (1.32 µm) lasers with their harmonics in visible and UV wavelengths. The third harmonics of the Nd laser in uv at 0.352 µm has been well developed by the laser fusion program of DOE, and the same technology can be used for a solar Nd laser when uv irradiation is needed. Since these laser systems utilize only a part of the solar spectrum, and the balance is rejected as heat, they are adaptable for cogeneration or a topping/bottoming cycle.
The new applications already proposed for concentrated solar photons, such as destruction of hazardous waste, production of renewable fuel (hydrogen by water photolysis), production of fertilizer, and air/water pollution controls, may benefit from the use of inexpensive solar pumped lasers matched with the photochemical kinetics of these processes. The benefits of using lasers will be an enhanced system efficiency and the discovery of new processes.