IV
Aircraft Operations
Consideration of aircraft operations, including inspection, maintenance, and repair procedures is crucial in the development and application of new materials and structures. This part of the committee's report focuses on the operation and monitoring of materials and structures in a service environment.
This part is organized in two chapters:
-
Chapter 7, "Aircraft Maintenance and Repair," describes the issues related to maintenance of commercial transport aircraft. The lessons learned from the aging of metal and composite structure are discussed.
-
Chapter 8, "Nondestructive Evaluation," describes current aircraft inspection practices and identifies needs for improved nondestructive evaluation techniques and promising technologies for the future.
7
Aircraft Maintenance and Repair
The successful utilization of new materials and structural concepts relies on maintenance programs that cost-effectively ensure passenger safety. This chapter is an overview of the current experience in aircraft maintenance programs, including inspection and repair processes, lessons learned from aging aircraft, and future needs to support new materials and structural concepts.
AIRLINE MAINTENANCE
Maintenance programs are evolved and developed for each new type of aircraft based on previous experience with similar materials, engines, components, or structures. New materials or structures, for which experience is limited, are observed more frequently until a basic level of confidence is established. Time extensions to inspection intervals are based on observations made during routine service checks. A typical airline maintenance and service plan is outlined in table 7-1. The objectives of an effective maintenance program are as follows (Edwards, 1994):
-
Ensure, through maintenance activity, that the inherent safety and reliability imparted to an aircraft by its design are sustained.
-
Provide opportunities to restore levels of safety and reliability when deterioration occurs.
-
Obtain information for design modification when inherent reliability is not adequate.
-
Accomplish the above at the lowest possible cost.
Structural Maintenance
Any new aircraft program is based on assessing structural design information, fatigue and damage tolerance evaluations, service experience with similar aircraft structures, and pertinent test results. Generally, the maintenance task evaluates sources of structural deterioration including accidental damage, environmental deterioration, and fatigue damage; susceptibility of the structure to each source of deterioration; the consequences of structural deterioration to continuing airworthiness including effect on aircraft (e.g., loss of function and reduction of residual strength, multiple-site or multiple-element fatigue damage, the effect on aircraft flight or response characteristics caused by the interaction of structural damage or failure with systems or power plant items, or in-flight loss of structural items); and the applicability and effectiveness of various methods of detecting structural deterioration, taking into account inspection thresholds and repeat intervals.
Component Maintenance
The application of new materials will not cause undue maintenance difficulties or hardship for the airlines provided the aircraft designer is familiar with component experience. Airline experience indicates that hardware items wear out, but statistical old-age wear-out in complex mechanical, electrical, and avionic components is not a dominant pattern of failure. In fact, over 90 percent of generic part types show either random distribution of failure or gradually increasing probability of failure with age (Edwards, 1994).
The reliability of a part or component of aircraft hardware is only as good as its inherent design (supported by adequate maintenance) allows it to be. Hence, it is generally accepted that (1) good maintenance allows parts to reach their potential reliability; (2) overmaintaining does not improve reliability, but does waste money; and (3) undermaintaining can degrade reliability. In general, fundamental design changes are required to correct inherent component reliability problems.
There are three approaches to preventative maintenance that have proven to be effective. The first method, hard time, involves removing a unit from service when it reaches a pre-ordained parameter value. The second method, functional check or inspection, involves monitoring a characteristic dimension or usage/operating parameter of a piece of hardware to determine if it is still suitable for continued operation, or if it should be removed to prevent an in-service failure. The third method, functional verification, requires performing an operational check of hardware function(s) to determine each function's availability if it is normally hidden from the scrutiny of the flight and operating crew.
There are many components for which measurement of deterioration, periodic removal for maintenance, and hidden function verification are not economically feasible or beneficial. Such parts require routine performance or reliability
TABLE 7-1 Typical Airline Maintenance and Service Plan
When Service is Performed |
Type of Service Performed |
Impact on Airline Service |
Prior to each flight |
''Walk-around"—visual check of aircraft exterior and engines for damage, leakage, and brake and tire wear |
None |
Every 45 hours (domestic) or 65 hours (international) flight time |
Specific checks on engine oils, hydraulics, oxygen, and specified unique aircraft requirements |
Overnight layover service |
Every 200–450 hours (22–37 days) flight time |
"A" check—detailed check of aircraft and engine interior, services and lubrication of systems such as ignition, generators, cabin, air conditioning, hydraulics, structure, and landing gear |
Overnight layover service |
Every 400–900 hours (45–75 days) flight time |
"B" check (or "L" check)—torque tests, internal checks, and flight controls |
Overnight layover service |
Every 13–15 months |
"C" check—detailed inspection and repair program on aircraft engines and systems |
Out of service for 3–5 days |
Every 2 years (narrow-body aircraft) |
Inspection and reapplication of corrosion protective coatings |
Out of service up to 30 days |
Every 3–5 years |
Major structural inspections with attention to fatigue damage, corrosion, etc. Aircraft is dismantled, repaired, and rebuilt. Aircraft is repainted as needed |
Out of service up to 30 days |
monitoring, and no preventive maintenance is required or desirable. Modern aircraft are more tolerant of failures than older aircraft designs because of the increased redundancy provided in the design.
Generally, most airlines classify specific component maintenance tasks as follows:
-
lubrication or servicing, where the replenishment of the consumable reduces the rate of functional deterioration;
-
operational or visual check, where identification of the failure must be possible;
-
inspection or function check, where reduced resistance to failure must be detectable and the rate of reduction in failure resistance must be predictable;
-
restoration, where the item must show functional degradation characteristics at an identifiable age, have a large proportion of units survive to that age, and be able to be restored to a specific standard of failure resistance; and
-
discard, where the item must show functional degradation characteristics at an identifiable age, and a large proportion of units are expected to survive to that age.
Malfunctions of components should be evident to the operating crew, have no direct adverse effect on safety (whether they occur as a single or multiple event), and minimize the effect on the operation of the aircraft itself.
SERVICE EXPERIENCE
Effective application of new materials on commercial aircraft requires the designer to consider potential sources of damage or degradation in operating environments and to develop a maintenance and repair approach to address them. Damage may occur due to flight loads, thermal and environmental cycles, and aircraft operation and servicing activities. A number of valuable lessons have been learned from
previous experience with metallic and composite structure in the current fleet. These lessons provide evaluation criteria in the application and servicing of new materials and structures.
Ramp and Maintenance Damage
An International Air Transport Association survey estimates that 36–40 percent of damage to aircraft is from ramp and maintenance damage, sometimes called friendly foreign object damage (IATA, 1991). Figure 7-1 shows a diagram of the Boeing 777 aircraft interfaces with servicing and other equipment (Boeing, 1994b). These areas are especially prone to damage and require robust material performance in these locations.
To determine the extent of groundhandling damage, 11 airline operators were queried for ground damage history during the years 1990 to 1993 (Boeing, 1994a). Of the 2,241 incidents reported, more than a third were from unknown causes. A tabulation of the causes of damage is given in table 7-2.
Ramp and maintenance damage can represent significant costs to the airlines. The repair of a damaged component is only part of the cost. The airline also bears the cost of flight delay or cancellation and the effects on connections and aircraft rotations.
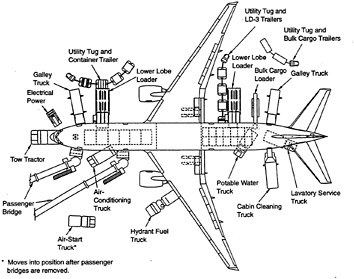
FIGURE 7-1 Diagram of aircraft interfaces with servicing and other equipment.
Source: Boeing (1994b).
TABLE 7-2 Causes of Ground Damage to Aircraft
Cause of Failure |
Number of Incidences |
Unknown |
773 |
Catering |
137 |
Belt loader |
122 |
Loader |
101 |
Lavatory and water service |
66 |
Container |
44 |
Jetway |
246 |
Baggage cart |
127 |
Tug/towbar/taxi |
109 |
Maintenance |
86 |
Cargo loading |
50 |
Fueling |
33 |
SOURCE: Boeing (1994a). |
Aging Aircraft
In April 1988, an Aloha Airlines Boeing 737-200 experienced an in-flight structural failure in which a large section of the upper fuselage ripped open and separated from the aircraft. The failure resulted from multiple-site damage (MSD) and corrosion. In this case, MSD was the link-up of
small fatigue cracks extending from adjacent rivet holes in a longitudinal lap joint in the fuselage. The accident focused international attention on the problems of operating an aging commercial fleet.
In 1990 approximately 46 percent of the U.S. commercial air transport fleet was over 15 years old, and 26 percent was over 20 years old. If current usage and replacement trends continue, the number of aircraft over 20 years old will double by the year 2000. Currently some 3,200 aircraft are affected by FAA Airworthiness Directives that concern operation and maintenance of the aging fleet. The review of experience with aging aircraft has caused an increase in the emphasis on stress corrosion, corrosion, fatigue, and MSD issues. This experience has caused, in turn, the selection of new aircraft alloys with better constituent chemistry control or changes in heat treatment tempers. Also, it has stimulated the development of new organic finishes that significantly retard corrosion, as well as the implementation of design practices to vastly improve corrosion resistance.
The FAA and NASA developed a cooperative research effort aimed at providing a technological basis for ensuring the continued safe operation of the aging commercial aircraft fleet. Each agency developed a program consistent with its mission. The FAA's National Aging Aircraft Research Program addresses the aging aircraft structural safety concerns and provides certification authorities and operators with the tools to meet those concerns. NASA's Airframe Structural Integrity Program is focused on developing advanced integrated technologies to economically inspect for damage and to analytically predict the residual strength of older airplanes. Together these programs form the technological basis for a cooperative effort with U.S. industry to address the critical aging aircraft issues.
Multiple-Site Damage
MSD is a form of widespread fatigue damage that is characterized by small cracks emanating from structural details such as fastener holes (Sampath, 1993). If cracks emanate from adjacent fastener holes, they have the potential to link up and lead to unexpected catastrophic failures as described in the previous section. Also, even without link-up, multiple-site cracks can severely degrade the capability of the structure to withstand major damage from other discrete sources as is described later in this section.
In the past, the standard industry practice was to visually inspect the airframe for damage. Various levels of inspections ranging from daily walk-around inspections to detailed tear-down inspections were performed. Instrumented nondestructive evaluation (NDE) methods such as eddy current probes were used only to inspect local regions of the structure where previous cracking problems had occurred. While these inspection methods were labor intensive and highly subjective, they were acceptable because the airframe was designed to survive a two-bay skin crack with a severed frame or stiffener. This design criterion was established to enable the airplane to tolerate major discrete source damage (i.e., such as might be encountered as a result of an engine structural failure) as well as large cracks resulting from the link up of smaller fatigue cracks or the unstable propagation of manufacturing flaws or other service-induced damage. Such damage is large enough that it should be easily detected, and the operator does not need to search for small cracks to ensure the structural integrity of the airframe. However, this "fail-safe" philosophy assumed that the structure adjacent to the major damage (e.g., the two-bay crack) was free of MSD. Design residual strength requirements were based on this assumption. However, the existence of very small cracks (e.g., a few hundredths of an inch or tenths of a millimeter in length) in the adjacent structure can severely degrade this residual strength and thus jeopardize the safety of the airplane as it did in the Aloha Airlines incident. Therefore, inspection of aging aircraft has become much more onerous than for newer aircraft because safety is vitally dependent on the detection of the very small cracks associated with this onset of MSD. This represents a major challenge to the inspection and aircraft industries.
The principal technical needs are (1) to develop and verify advanced NDE technology that can reliably and economically detect disbonds, small MSD fatigue cracks, and corrosion and characterize their effect on the residual strength; and (2) to develop and verify advanced fracture mechanics and structural analysis methodology to predict fatigue crack growth and residual strength of airframe structures to determine in-service inspection thresholds and repeat intervals, quantitatively evaluate inspection findings, and design and certify structural repairs. NDE methods related to MSD are described in chapter 8, and fracture mechanics and structural analysis methods are described in chapter 6.
Corrosion
Corrosion of aging aircraft has been described as an insidious problem (Marceau, 1989). While other aging mechanisms, such as wear and fatigue, are somewhat predictable and can be addressed by the airline maintenance programs to preclude major structural problems, corrosion—especially in its localized forms—is very difficult to predict and detect. Factors that influence the extent of corrosion on aircraft are materials selection, design, component processing and finishing, operational environments, and maintenance programs.
It is anticipated that airplanes manufactured today will experience fewer corrosion problems than those in the current aged fleet because of significant design and corrosion protection improvements that have been implemented and because of operators' increased awareness of the role of these improvements in preventive maintenance. Clearly, maintenance
and corrosion control programs will continue to play a major role in the control of corrosion as airplanes age.
Some examples of design improvements to reduce corrosion on the Boeing 777 (Marceau, 1994) include:
-
enhanced drainage, especially in the keel of the aircraft;
-
the sealing of faying surfaces in corrosion-prone areas;
-
the application of improved finish systems;
-
liberal use of corrosion-preventive compounds;
-
implementation of a good corrosion control maintenance program; and
-
improved access for inspection of corrosion-prone areas.
Major airline fleets include aircraft ranging in age from new to 25 years old. Consequently, the degree of corrosion protection incorporated into the airplane varies from limited protection for older aircraft to fairly extensive protection for newer aircraft. Corrosion control programs are tailored to individual fleets, depending on age, prior experience, flight environment and degrees of corrosion protection incorporated prior to the delivery of the aircraft (DeRosa, 1995). All protective finishes are maintained and corrosion prevention compounds are applied during periodic maintenance. Critical areas that are prone to excessive corrosion include areas below the galleys, doorways, lavatories, cargo compartment subfloors, inside external fairings, and the bilges which are all treated at four-year intervals. Landing gear wheel wells and wing spars are treated yearly. Longer intervals of time are allowed between reapplications of corrosion prevention compounds in the case of less-severe environments.
Aging aircraft repairs have typically involved upper-skin lap fastener replacement, nonbonded skin panel replacement, skin lap doubler repairs, frame reinforcement, entryway door and scuff-plate doublers, replacement bushings and clevis joints, bulkhead forging replacement, and selected landing gear component replacement. Based on service experience, the airlines have expectations that manufacturers of new aircraft will (DeRosa, 1995):
-
include stress corrosion prevention in all design reviews with airline customers,
-
assemble all structure below the aircraft floor with sealant on all faying surfaces,
-
coat all detail parts with corrosion-inhibiting primer or polyurethane topcoat before assembly,
-
not use adhesive-bonded fuselage skin panels below the floor line or in areas subject to severe corrosion environments such as galleys and lavatories,
-
treat all basic fuselage structure with corrosion-preventive compounds, and
-
perform a complete (100 percent) inspection for delamination of bonded skin panels prior to the aircraft delivery in order to establish a baseline for subsequent inspection.
The objective of aging aircraft programs is to ensure the continued airworthiness of large transport aircraft as long as they remain in commercial service (Curtis and Lewis, 1992). Because new materials and fabrication processes may yield different degradation and damage mechanisms, a preproduction review should ensure that the new aircraft design includes lessons learned from the existing aging fleet.
Many of the steps needed to improve aging performance are detailed below. Most of these steps have now been incorporated into recent aircraft designs. The susceptibility of aircraft to corrosion and MSD fatigue can be reduced by the following steps:
-
eliminating cold-bond lap-joint design details;
-
providing adequate drainage to eliminate corrosion in areas where moisture accumulates;
-
using the most corrosion-resistant materials and tempers available;
-
evaluating galvanic couples with typical coating damage;
-
testing dissimilar materials design details for size effects in areas with joints;
-
controlling design stress levels, improving design details, and utilizing improved manufacturing and maintenance procedures to preclude the onset of multiple-site damage within the operational lifetime of the airplane;
-
developing predictive and monitoring techniques for the onset of MSD (simple and cost-effective techniques should be integrated into the aircraft maintenance plan); and
-
providing a complete corrosion prevention and control program within the aircraft maintenance program upon delivery of an aircraft.
The present focus on aging aircraft will lead to better corrosion-resistant treatments for next-generation aircraft. Materials selection in wet areas, the design drainage schemes, the use of insulation standoffs, and sealing and finishing systems have all been improved. The benefits of these improvements should be evident during in-service performance of the Boeing 777 and future aircraft. Liberal use of corrosion-preventive compounds applied in the aircraft assembly process and periodically in service, using a good corrosion control maintenance program, should minimize future corrosion concerns.
Structural Composites
As discussed in chapter 4, prior to the latest generation of aircraft, which includes the Airbus A320 and the Boeing 777, structural composites have been used on aircraft flight control surfaces such as elevators, spoilers, ailerons, and rudders, as
well as fairing and fillet panels, landing gear doors, engine cowl doors, and other secondary structures. For these applications, honeycomb sandwich designs with thin 0.6—1.5 mm (0.024—0.060 in.) composite facesheets are most common. It follows that most of the experience with advanced composites has been obtained with this kind of construction. Previously, similar constructions with fiberglass skins and nonmetallic honeycomb core have been used. There is much less service experience with thicker-skin laminate designs that have been used in composite primary structure.
In general, the service experience with composites indicates that damage occurs because of discrete sources such as impacts, lightning strikes, and handling rather than progressive growth caused by a fatigue condition (Blohm, 1994). In addition to groundhandling damage, a recent survey by the International Air Transport Association, summarized in table 7-3, lists the particular causes of damage that occur in the current generations of composite structure (IATA, 1991).
The types of damage to composite components include disbonds or delaminations (45 percent), holes or punctures (35 percent), cracks (10 percent), and other damage (10 percent). An especially difficult maintenance issue resulting from these types of damage is when perforation allows the incursion of hydraulic fluids, water, and other liquids into the honeycomb core. Composites may also suffer loss of load-bearing capability due to resin charring and the potential for corrosion of adjacent metallic surfaces. Typical causes of composite service damage mechanisms are shown in table 7-4.
Service experience with thicker composite laminate constructions, such as that used on primary structures on the Airbus A320 and Boeing 777, is not adequate enough to establish damage trends.
Composite Repair
The current methods used by the airlines to repair damage to aircraft composite structure (secondary structure and primary flight controls) depend on the extent of damage, the time available to perform the repair, and the time until the next scheduled maintenance visit. In approximately 80 percent of all cases, the damage is covered with adhesive-backed aluminum foil ("speed tape") or temporarily repaired and deferred for a specific time to provide for interim or permanent repair or part replacement. Occasionally, temporary or permanent repairs can be performed by bonding or bolting a sealantcoated metal or precured composite overlay over the damage. Finally, most permanent repairs are accomplished with room-temperature curing, wet lay-up and precured patch techniques. Other permanent repairs use prepreg that cures under vacuum or autoclave pressures at temperatures lower than the cure temperature of the original structure. Repair resins are being developed that have relatively low cure temperatures,
TABLE 7-3 Most Common Causes of Composite Structure Damage to Aircraft
Cause of Failure |
Percent of Incidences |
Moisture and chemical fluids attack |
30 |
Other (heat damage, fatigue, abrasion, and erosion) |
11 |
Bird strikes and hail damage |
8 |
Runway rocks and foreign object damage |
8 |
Lightning strikes |
7 |
SOURCE: IATA (1991). |
but have thermal and environmental resistance similar to higher-temperature curing systems.
The thicker laminate construction used in composite primary structure are not conducive to wet lay-up patch technologies. Thin facesheets on honeycomb panels are currently repaired using bonded scarf patches with a scarf taper of 20:1. For thicker constructions the result would be the removal of a large amount of undamaged material (Bodine et al., 1994). The emphasis in the development of primary structure repairs has therefore been on fastened, precured composite or metallic splice plates, similar to current metal repair techniques. A design for a fairly complex bolted repair is shown in figure 7-2. The issues that must be addressed in these types of repairs include (1) criteria for determining when repairs are required; (2) availability of standardized repair elements; (3) drilled hole quality; (4) ability to restore original strength, durability, and damage tolerance; and (4) ability to match existing contours.
Given typical flight schedules, composite structure repair must be accomplished within eight hours on an overnight layover, otherwise the part would be replaced with a spare. Repairs carried out during an overnight stop (at line stations or hubs) and repairs requiring more-intensive maintenance center rework should follow guidelines established by the manufacturer's structural repair manual and the appropriate industry group, the Commercial Aircraft Composite Repair Committee. Because composite structures are fabricated from a large number of resin/fabric systems from several qualified suppliers worldwide, it is difficult and expensive for airlines to stock a wide variety of repair materials. Accordingly, there is a pressing need for standardization of repair materials and processes.
While next-generation aircraft are expected to use laminated or tailored composite skin structure that is more damage tolerant, there are many lessons that have been learned in maintaining and repairing the thin-skin, nonmetallic honeycomb sandwich constructions on today's aircraft that need to be considered in new aircraft design and materials selection. Maintainable designs need to consider component accessibility, permitted defect levels, and nondestructive testing
TABLE 7-4 Causes of Service Damage to Composite Structure
Damage Mechanism |
Affected Components |
Mechanical Damage (in flight) |
|
Hail impact |
Radome Engine inlet Upper wing and tail plane fixed panels Flight controls |
Bird strike |
Engine inlet cowl Radome |
Engine disintegration |
Engine cowl Fuselage Lower wing and tail plane fixed panels |
Tire protector separation |
Flaps Lower wing-to-body fairings Landing gear doors |
Mechanical Damage (on ground) |
|
Hail impact |
All horizontal surfaces (wing panels, flight controls, upper areas of engine cowl) |
Groundhandling equipment |
Engine cowl Wing and tail leading and trailing edges Landing gear doors |
Mishandling |
Engine cowl Access doors |
Overload due to actuation system failure |
Flight controls Spoilers Thrust reverser |
Transport and handling |
All removable components |
Lightning strike |
Radome Leading and trailing edge components (aileron, rudder, elevator, leading-edge fairings) Engine cowl |
Overheat |
Engine cowl Landing gear door (in case of brake overheat) |
Erosion |
Radome Engine inlet cowl Leading-edge fairings |
Chemical contamination |
|
Skydrol (hydraulic fluid) leakage |
Engine cowl Actuated components (flight controls, spoilers) |
Paint stripper |
All painted components |
Corrosion |
All aluminum honeycomb with composite faceskins Improperly isolated aluminum brackets, hinges, etc. |
SOURCE: Blohm (1994). |
techniques. Nondestructive test indications need to be correlated with structural criteria throughout the life of the aircraft.
composites must be protected by finishes with resistance to fluid penetration and ultraviolet degradation. significant costs are incurred by the airlines over the life of an aircraft in the maintenance of protective finishes. The durability of protective finish systems (including aerodynamic surfaces) should be characterized prior to production.
The removal of finishes from composites is a slow and expensive process. Since chemical strippers attack the polymer matrix, airlines generally remove finishes through mechanical abrasion processes. New paint removal processes like laser, heat, frozen carbon dioxide blasting, and wheat starch blasting are being evaluated. Rapid, low-cost, on-aircraft paint removal techniques require implementation if larger areas of composite surfaces are to be accepted on the next-generation aircraft.
Fast, durable, temporary, and permanent field and shop repair procedures should be developed for new composites. This would include development of repair materials, tooling, and processes for high-modulus, high-strength composite skins, metallic and nonmetallic honeycomb sandwich structure, and laminated hybrid and bonded metal structures. These repairs should be accomplished without major disassembly of structure—preferably while working from an exposed exterior or interior surface. It is critical to design for accessibility and interchangeability of parts, especially those for parts that have high damage probability.
SUMMARY
Consideration of aircraft maintenance and repair procedures is a critical part of the development and application of new materials and structures. Previous service experience with metallic and composite structures supports the importance of a maintainable design. The experience of the aging fleet with metallic structures provides lessons in corrosion prevention and control as well as detection and control of multiple-site fatigue damage through appropriate analysis methods, improved component designs, and focused inspection and maintenance. Experience in thin-skin composite components suggests emphasis on robust and durable component design and standardization of repair criteria, materials, and procedures.
Additional experience suggests that the heavy dependence on the costly and intensive inspections to maintain safety in aging airplanes must be reduced further by implementing new and easier to use inspection techniques; utilizing materials with better damage tolerance, toughness, and visible warnings of impending failure; and designing components with adequate access for inspection and maintenance to preclude hidden defects. The reliance on inspection technology can also be reduced by designing structure that is tolerant of
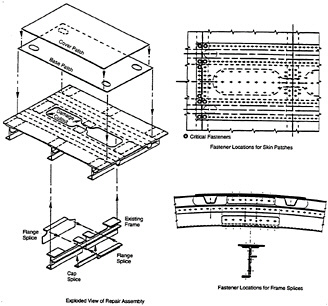
FIGURE 7-2 Bolted splice repair of a composite primary structure panel. Source: Bodine et al. (1994).
undetectable defects or damage; establishing damage limits as well as specific inspection standards and techniques; and developing damage-tolerant, fatigue-resistant structural repairs for new materials along with the means for inspection validation.
The FAA should work with the airline and manufacturing industries to develop common standards and procedures for maintenance and repair of aircraft structure. Application of new materials, processes, and component designs need to be anticipated and accounted for in the FAA's research priorities.