2
Scientific Background
Although the Sun has been studied for centuries, it is only since the advent of the space age that its complexity and the full range of its effects on Earth and other planets in our solar system have been fully appreciated. For example, sunspots can be seen with the naked eye and were observed in ancient times, but only since the advent of the telescope are the 11-year cycles accurately chronicled in human records, and it has been only 3 or 4 cycles since the discovery that Earth is continuously bathed in a supersonic “wind” of ions, electrons, and a magnetic field streaming incessantly from the Sun. At about the same time, researchers became aware of the magnetic bubble of the magnetosphere surrounding Earth, which deflects most of the solar wind around our atmosphere. The highly variable solar wind compresses, stretches, and buffets this magnetic shield constantly. In the early 1970s, Skylab images of the Sun revealed both x-ray dark regions (“coronal holes”) that give rise to high-speed streams, as well as huge eruptions traveling outward through the solar atmosphere. Measurements from the Solar Maximum Mission showed that even the output of the Sun is not constant. Although the strongest variations occur in the normally weak invisible parts of the emitted electromagnetic spectrum, Earth 's atmosphere still “detects” and responds to these. The nature and extent of that response remain subjects of investigation.
One of the most striking features of our Sun is its cycle of “activity.” The numbers of sunspots, solar flares, solar radio bursts, and coronal disturbances increase and then decrease again every 9 to 13 years. Only during the last three cycles has it been generally appreciated that the underlying driver of this cycle is the solar magnetic “ dynamo,” a convecting layer of electrically conducting material inside the Sun that produces a surface magnetic field of changing character with nearly periodic behavior. These types of cycles have now been remotely sensed on other solar-type stars in our galaxy.
The solar cycle seems to be linked with a wide range of cyclic changes in the geospace environment—for example, changes in the upper atmosphere, the ionosphere, the cosmic-ray-related radiation levels, Earth's surface magnetic field, and the Van Allen radiation belts. Geospace is coupled to the Sun, and as inhabitants of Earth and members of a technologically advanced society, we are compelled to understand the causes, modes, extent, and consequences of that coupling.
The coming maximum in solar activity raises two central questions with basic scientific and applied aspects:
-
How does the Sun undergo its dramatic changes during the rise to solar maximum?
-
What are the practical consequences for Earth and our society?
Magnetic fields generated by the solar dynamo can only be directly observed at and above the visible surface where sunspots contain the strongest concentrations of field. The solar activity cycle was first noticed in changes of the number, pattern, and magnetic polarity of the sunspots over the average 11-year cycle. Even early observations hinted that the fusion processes producing radiant energy deep within the Sun were quite steady, although the interaction of the solar field with its outer layers was not. Images of the Sun taken through filters that isolate narrow ultraviolet (UV), extreme ultraviolet (EUV), soft x-ray, and optical
wavelength bands show that the fields extend far above the sunspots, producing sometimes complex patterns of loops and arcades. These images also indicate the presence of weaker magnetic fields between the sunspots that underlie larger-scale systems of loops and arcades visible in the corona during solar eclipses.
Most frequently at solar maximum, the interactions between the magnetic fields and the moving, ionized solar atmosphere can twist strong fields above sunspot groups into unstable configurations storing substantial amounts of energy. This energy can be suddenly released in solar flares, which temporarily enhance the solar radiant output at radio and x-ray wavelengths by several orders of magnitude. The larger-scale magnetic structures also undergo frequent eruptions during solar maximum. The ejecta from these coronal mass ejections (CMEs) can travel outward through the background solar wind at speeds of up to 2,000 km/s. These fast CMEs cause interplanetary shocks as they move, thereby greatly enhancing the density and fields in the solar wind. The large-scale character of solar magnetic fields over the cycle also determines the large-scale pattern of coronal fields carried by the solar wind.
Although the solar magnetic field is considered ultimately responsible for all solar variability, the physics underlying its character is not yet understood. Models of the solar dynamo are able to simulate a cyclical behavior, but the changes they predict are inconsistent with observations. This problem seems to stem in large part from an an inaccurate description of the dynamics of the solar interior. Helioseismology techniques now allow researchers to probe the interior structure and dynamics of a star. In just the last few years it has been learned that the differential solar rotation observed on the surface persists inside the visible Sun to about two-thirds of its radius from the center, where there is a strong, thin shear layer. Inside that radius, the Sun rotates almost as a solid body. The implications of this new knowledge are just beginning to be explored but raise even more important questions: Does the situation change in any way as the solar activity cycle progresses? Are the origins of sunspots and CMEs apparent beneath the surface? Do the magnetic fields ever become dynamically important in the solar interior? Is this a critical factor in explaining the Sun's activity cycle? The intensity of the solar maximum varied dramatically over time. What aspect of the solar dynamo determines what the solar maximum intensity will be? What are the consequences for Earth?
EARTH'S SPACE ENVIRONMENT RESPONSE
Only since the last solar maximum have researchers begun to understand the fundamental geospace responses to the maximum and minimum of solar activity. Even the limited spacecraft capabilities that were available for solar and space environment observations during the last solar maximum resulted in a revolution of scientific understanding of the causes and consequences of geomagnetic activity. It is now clear that conditions in interplanetary space and geospace can become extreme and highly dynamic.
In particular, the Van Allen radiation belts, previously regarded as slowly varying and predictable features, were found to be much more dynamic and transient than any statistical model would imply. The bulk of the major geospace disturbances were a consequence of the CMEs, which on occasion compress the magnetosphere to altitudes well inside the geosynchronous orbit (at 6.6 Earth radius [RE equatorial). Those CMEs that travel fast enough with respect to the low-speed (~400 km/s) ecliptic solar wind, moreover, produce leading shock waves that were a major cause of solar energetic proton events. These events can contribute to
the radiation belt for weeks or months, adding to other transient populations that follow the passage of the shock front through the magnetosphere. Moreover, by virtue of their disturbance of the entire magnetosphere, it is the CMEs that cause widespread effects including auroral activity, ionospheric disturbances, and induced ground currents.
Further discoveries have resulted from the observational capabilities that became available during the present solar minimum period. These illustrated the special role of high-speed solar wind streams emanating from coronal holes during solar inactive periods. These high-speed wind streams somehow control the intensity of energetic (>1-MeV) electrons in the radiation belts with a fair degree of predictability.
We are now witnessing a transition from the high-speed solar wind stream activity characteristic of a solar minimum to the less predictable eruptive events that characterize a solar maximum. This change in behavior manifested itself most spectacularly with the event of January 6-11, 1997, when a CME produced an enormous magnetic cloud that expanded toward Earth. The CME produced an interplanetary shock that hit the magnetosphere on January 10, shortly after midnight Universal Time. The shock was followed roughly 24 hours later by an interval of unusually high-density solar wind (150 cm−3, roughly 15 times the average solar wind density) that compressed the magnetosphere on the dayside within the geosynchronous orbit. This strong pressure pulse was followed by a stream of higher than average speed (~600 km/s). A rapid buildup within the inner magnetosphere of energetic (>1 MeV) electrons by 3 to 4 orders of magnitude was under way before arrival of the high-density solar wind impulse. Figure 1 illustrates the connections that can now be made between solar, interplanetary, and geospace observations during such events.
What was particularly significant about this January 6-11, 1997, event was the first-ever opportunity to observe the effects of a CME from cradle to grave: Observations from the Solar and Heliospheric Observatory (SOHO) and Yohkoh showed the changes in the photosphere and corona that preceded the eruption. The coronagraph on SOHO then imaged the dense plasma accompanying the erupting fields, the CME, as it moved away from the Sun. Radio waves emitted from the approaching shock front were detected on board the Wind spacecraft (as was the passage of the leading interplanetary shock and the trailing density pulse), while the Polar spacecraft and other magnetospheric satellites and ground-based observatories measured the shock's effects on geospace. Theory teams sponsored by the International Solar-Terrestrial Physics (ISTP) program produced a global numerical simulation of the event, the longest to date, to capture the entire period of magnetic cloud passage and to analyze the physical causes of the energetic particle increases and atmospheric effects. Researchers have never been so well prepared to study the complete life cycle of this type of complex and important natural event.
EARTH'S CLIMATE RESPONSE
The past 15 years have also produced explosive growth in our knowledge of our Sun's outputs and the responses of Earth's atmosphere to them. Accumulating records of the total solar radiative output1 are finally yielding accurate measures of the degree to which the “solar constant” actually varies (by ~0.1%) as the contemporary Sun goes through the extremes of its ~11-year activity cycle. In addition, images of the Sun in wavelengths over a broad range of the
1 |
Board on Global Change, National Research Council, Solar Influences on Global Change, National Academy Press, Washington, D.C., 1994. |
electromagnetic spectrum (of which SOHO's new full-disk images, shown by examples in Figure 2, are especially remarkable) allow for determination of what very different regions of the solar surface dominate the various spectral lines and bands as well as how historical proxies (such as sunspot number) can be used to infer much longer-term solar radiative behavior.
As noted above, significant progress has been made in understanding the solar cycle and shorter-term variations of the particle and field outputs of the Sun, including the solar wind and solar energetic particle fluxes. The different consequences of flares and CMEs on the Sun, in interplanetary space, and at Earth are beginning to be understood.2 Yet the physical means by which these different aspects of solar variability affect Earth's atmosphere are as diverse as their own underlying solar sources. Some remain controversial and others await validation at a time when an unprecedented combination of state-of-the-art models and instrumentation can be used for the first time during a period of maximum solar activity.
Several examples serve as the best illustration. Research using less comprehensive and sensitive data sets than are available for the upcoming solar maximum has established that the magnitude of total solar irradiance variations is determined largely by a combination of the brightening of faculae (bright, patchy areas seen in monochromatic light that surround sunspots and active regions) partially canceled by sunspot blocking. However, how the Sun's magnetic field establishes the degree of imbalance of these two magnetically controlled features remains unknown. Moreover, another significant contribution may come from the “active network,” a region on the visible Sun's surface that has been inadequately characterized. These total irradiance variations are the solar variations most likely to directly affect Earth's climate over long time frames.3 If it can be learned how solar magnetic fields modify the solar constant by quantifying the amount of sunspot blocking compared to facular and network brightening (e.g., with the tools that SOHO and Yohkoh provide), researchers may be able to interpret features in Earth's climate record such as the Little Ice Age, which coincided, remarkably, with a period of low sunspot numbers known as the Maunder Minimum. It might also be possible to make more extensive estimates of such effects over history, to better use solar-type star observations to infer Earth's distant past and its destiny, and to better extrapolate to conditions on the other bodies in the solar system and around other Sun-like stars.
A second example relevant to the relationship between the Sun and Earth's climate concerns the possible role of the ionization state of Earth's atmosphere, which is known to affect its chemistry, its response to solar wind disturbances of the magnetosphere, and other processes that depend on the presence of free charges. Although this state is mainly controlled by the solar UV and EUV flux responsible for the ionosphere proper, some deeper ionization is produced by solar x-rays, solar energetic protons, and galactic cosmic rays. The flux of galactic cosmic rays at Earth and other solar system bodies is known to be reduced during periods of high solar activity. At the same time, the more variable fluxes of generally less energetic particles from solar flares and from CME-driven interplanetary shock acceleration of some solar wind particles increase greatly. The flares also produce impulsive bursts of energetic electromagnetic radiation (UV, x-rays, and sometimes gamma rays). These different energetic emissions all increase the amount of ionization in the atmosphere.
2 |
Space Studies Board, National Research Council, Space Weather: A Research Perspective, 1997. This report is not available in hard copy; it may be viewed on the World Wide Web at the following address: <http://www.nas.edu/ssb/cover.html>. |
3 |
Board on Global Change, National Research Council, Solar Influences on Global Change, National Academy Press, Washington, D.C., 1994. |
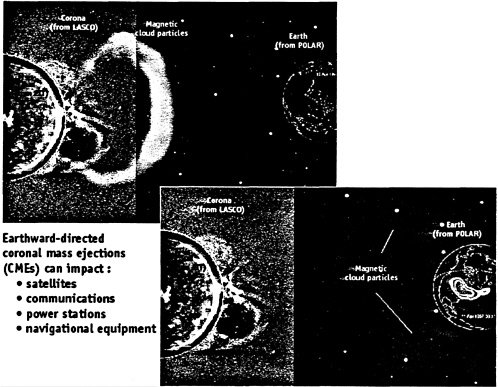
FIGURE 1 An Earthward coronal mass ejection is observed by the Extreme-ultraviolet Imaging Telescope (EIT) and Large Angle and Spectrometric COronagraph (LASCO) instruments on SOHO. Its effects on Earth's magnetosphere are analyzed by POLAR, a spacecraft in the International Solar-Terrestrial Physics program.
FIGURE 2 (facing page) This figure illustrates the variety of images of the Sun that are currently available, including new full-disk images from SOHO (clockwise from the upper left):
-
A SOHO Michelson-Doppler Instrument (MDI) magnetogram of the Sun.
-
An x-ray image of the Sun taken by the Japanese spacecraft Yohkoh.
-
An image of the Sun in the neutral helium emission line at 1,083 nanometers (He I) from the U.S. National Solar Observatory at Kitt Peak, Arizona.
-
An image of the Sun in the singly ionized helium emission line at 304 angstroms (HE II) from the SOHO EIT Telescope.
In the center of the figure are two images. The larger is SOHO's LASCO C3 instrument showing a blast of particles expanding through the Sun's corona. Superimposed where the Sun would be is a High Altitude Observatory coronameter image taken at Mauna Loa, Hawaii.
Although the geographical regions (e.g., polar for most of the particle fluxes except for the most energetic cosmic rays), times (e.g., daytime for solar flare UV and x-ray bursts), and altitude ranges affected are generally understood, the consequences are by no means yet known. Whereas the resulting electron density enhancements obviously affect our use of the ionosphere, there are known atmospheric chemistry effects (including stratospheric and mesospheric ozone chemistry perturbations and suggestions of possible influences on high-altitude clouds) that require more study. The cause-and-effect chain can be examined by modern cosmic-ray and solar particle detectors and sensitive atmospheric measurements (available in some cases from the newest experiments within the Earth sciences community). Indeed, the ionization link has consistently recurred as a possible explanation for solar variability effects on climate through some nonlinear process. No previous period of high solar activity has been so well endowed with facilities and resources for establishing or refuting the significance of such mechanisms.
The preceding discussion has broad implications beyond the study of space physics. For example, the consequences of solar variability on Earth lead us to ask how these variations affect other planets in our solar system. What happens on a planet like Mars, which lacks a protective magnetic shield or substantial atmosphere like Earth 's? How have Mercury's surface and atmosphere been altered over time by the variable solar irradiations at close range? To what extent are the giant planets and their satellites immune to “space weather”?