Molecular Biology and Biotechnology in Marine Toxicology
Mark E. Hahn and John J. Stegeman
THE PROBLEM
The presence of toxic chemicals in the marine environment has long been recognized as a potential threat to human health and to the health of the oceans. The oceans are the ultimate sink for many chemicals of anthropogenic origin but also are a source of naturally occurring toxins (and pharmacological agents). Certain classes of marine pollutants —especially persistent organic chemicals such as halogenated aromatic hydrocarbons—are globally distributed, occurring even in the most remote areas such as polar regions, the open ocean, and the deep sea (Ballschmiter and others 1997; Muir and others 1988; Stegeman and others 1986). In many coastal areas, the concentrations of chemicals in the environment are extremely high (e.g., Weaver 1984).
Marine pollutants have been classified primarily on the basis of their chemical structure (e.g., polynuclear aromatic hydrocarbons [PAHs]) or original source or use (e.g., petroleum hydrocarbons or pesticides). Increasingly, however, chemicals are being grouped by functional characteristics, i.e., properties related to shared effects or mechanisms of action (e.g., “endocrine disruptors” [Limbird and Taylor 1998; McLachlan 1993; NRC 1999]). The chemical nature and possible human health effects of some marine pollutants have been considered in earlier reports (Ahmed 1991).
Biology Department, Woods Hole Oceanographic Institution, Woods Hole, MA
The field of toxicology is concerned with the interactions of such chemicals with biological systems, from molecules to ecosystems. Toxicology truly is an integrative science (much like oceanography) and is rooted in established, basic disciplines from molecular biology and biochemistry, to physiology, and even to ecology. In many respects, an organism's response to chemicals in the environment is another facet of biochemical adaptation (Hochahka and Somero 1984; Prosser 1986). Much of the current effort in toxicology is aimed at understanding, at the most fundamental level, the mechanisms underlying chemical effects, which should bring the science from a descriptive to a predictive mode.
Research in marine toxicology ultimately seeks to understand, monitor, and predict the effects of contaminants. These three objectives are interrelated (Figure 1), and progress in meeting all three will be aided substantially by the use of molecular and biotechnological approaches. In turn, the understanding gained should help to shape the application of biotechnology in practical approaches to monitoring.
Two features of toxicology, and biological sciences in general, seriously aggravate the challenge to understanding and ultimately predicting the nature and significance of the interaction of pollutants with marine organisms. The first is the complexity of the problem. When one considers the number of chemicals that are of potential concern (104-105) and marine species that exist as possible targets (106-107 [May 1988; Pimm and others 1995]), it becomes obvious that achieving a satisfactory understanding will not be possible using a species-by-species or chemical-by-chemical approach.
Other sources of complexity include the fact that organisms are usually exposed not to single chemicals but to chemical mixtures, the components of which may interact in unexpected ways. We need to better understand and predict additive, synergistic, or antagonistic interactions between chemicals. We must also consider interactions between chemicals and environmental variables such as salinity, temperature, light, and pressure. Finally, we must consider chemical effects at multiple levels of biological organization.
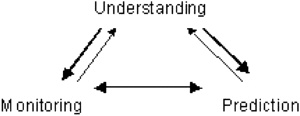
FIGURE 1. The three interrelated objects of marine toxicology research.
The second feature that contributes to the challenge of marine toxicology is variability. Marine systems are characterized by geographic and temporal heterogeneity in the concentrations of chemicals and in the structure of communities and ecosystems. In addition, species differences in sensitivity to contaminants and mechanistic variability across taxa complicate the extrapolation of results from one species to another.
CHALLENGES/RESEARCH NEEDS
Progress toward assessing the impacts of contaminants in the marine environment will require a more complete understanding of the interactions of these chemicals with biological systems. Such an understanding must occur at the level of molecular mechanisms as well as at higher levels of organization, including populations and ecosystems. It will require basic research to determine the general principles (unity) as well as the detailed differences (diversity) that affect our ability to extrapolate across species or systems.
A mechanistic understanding will stimulate the development of tools for monitoring marine organisms for exposure to and effects of chemical contaminants. Such research must go beyond the current approach of monitoring exposure by measurement of chemical residues in biota; the volume of such data has outstripped our ability to interpret it in a biologically meaningful way. It is necessary to move toward biologically based monitoring, by using mechanism-based biomarkers and bioassays (Hahn 2000; Stegeman and others 1992). In addition, monitoring long-term effects of contaminants at higher levels of biological organization will become increasingly important. For example, changes in the population structure or genetic diversity of exposed populations may reveal effects of greater significance for the ecosystem than those measured in individual animals within those populations (Guttman 1994; LeBlanc 1994).
A mechanistic understanding of chemical action and species differences in susceptibility, combined with appropriate monitoring tools, will facilitate the prediction of chemical effects (including identification of the most sensitive components of the ecosystem) and the consequences of remediation efforts. Thus, the process of ecological risk assessment will become more accurate, and therefore more useful and less controversial.
Thus, the challenge to marine toxicology, as in other areas of biology, is to extract from the complexity/variability an understanding of the common themes that underlie the responses of the organisms, and conversely to characterize and understand the diversity in organismal responses, and to determine the mechanistic bases for both. Addressing these important questions in marine toxicology can proceed only by basic research, taking full advantage of the advances in molecular biology and biotechnology.
APPROACHES AND EXAMPLES
In this section, we briefly describe some of molecular biotechnological approaches that will be essential to progress in meeting the three objectives outlined above; and in Figure 1, we illustrate their utility with examples drawn from research on halogenated aromatic hydrocarbons (HAHs), a major class of marine pollutants. Although we focus on HAHs, the approaches described will be useful for research on other contaminants as well.
Molecules and Mechanisms
Achieving a mechanistic understanding of chemical effects is a primary goal of research in marine toxicology. Included in this goal is the desire to understand, at a molecular level, the mechanistic basis for species differences in sensitivity to specific contaminants. Much of this information will come from the characterization of the genes—and their protein products—that are involved in toxicity.
An important area of inquiry in marine and aquatic toxicology has emerged from the recognition that many chemicals are toxic by virtue of their ability to interact with intracellular receptors, in some cases mimicking or blocking the effects of natural hormones (Colborn and others 1993; NRC 1999; Schmidt and Bradfield 1996). Toxicity occurs when the resulting changes in gene expression are spatially or temporally inappropriate. The list of such receptors known from studies in laboratory mammals is long, and growing (Table 1). In addition to receptors and other proteins involved in signal transduction, a variety of enzymes modulate the response of organisms to chemical exposure. This variety includes enzymes involved in biotransformation reactions (e.g., the cytochrome P450-dependent monooxygenases) as well as enzymes involved in protective functions (e.g., DNA repair). For each class of proteins, knowledge of their diversity, structure, and function in marine organisms is woefully inadequate relative to the need for such information to understand the impact of chemicals in marine systems (Table 2). For example, although the number of biotransformation enzymes known to exist in humans exceeds 100, fewer than two dozen of these have been studied in any marine organism, and even the most well-studied of these enzymes have been investigated in only a handful of species.
Two types of information will improve our understanding of receptors and enzymes and their role in the toxicity of chemicals to marine organisms. One type is knowledge of the comparative biochemistry and molecular biology of these proteins. Although progress has been made for some of these proteins (Hahn 1998a; Livingstone and Stegeman 1998; Stegeman and Hahn 1994), there is still much to learn (Table 2).
TABLE 1. Soluble Receptors Involved in Xenobiotic Effects
In investigating toxicologically relevant genes and proteins in marine species, we can expect some surprises in comparison with existing knowledge obtained in terrestrial mammals. Recent findings arising from studies on the mechanism of dioxin toxicity in marine organisms serve as an example. It is well known that the toxicity of chlorinated dioxins and related compounds occurs through activation of a transcription factor known as the aryl hydrocarbon receptor (AHR), and a single AHR gene has been identified in laboratory animals and humans (Schmidt and Bradfield 1996). Recently, a second, novel AHR gene was identified in Fundulus heteroclitus, an estuarine fish (Hahn and others 1997; Karchner and others 1999). This second AHR (AHR2) has since been identified in a variety of marine and freshwater fish, and differences in specific sequence motifs and in patterns of expression suggest that the two AHR forms could have distinct functions (Abnet and others 1999; Karchner and others
TABLE 2. Diversity of Genes Involved in Mechanisms of Toxicity: Lack of Information in Marine Speciesa
Gene class (examples) |
Number of genes per species (mammals) |
Number of genes characterized in marine animals (# species) |
Signal transduction (receptors) bHLH-PASb/Ah receptorb Nuclear receptors Neurotransmitter receptors |
>100 |
10 (30) |
Biotransformation Cytochrome P450s Flavin monooxygenases Transferases |
~100 |
15-20 (30) |
Repair/protection DNA repair Oxidoreductases Peroxidases Catalases |
~50 |
0 |
a The numbers listed are order-of-magnitude estimates of the numbers of genes and species for which information is available. These estimates illustrate the diversity of genes involved and the relative lack of information about these genes in marine organisms. bbHLH-PAS, basic-helix-loop-helix-Per-ARNT-sim; Ah, aryl hydrocarbon. |
1999). The exact roles of these two AHRs are not yet known but could be related to the sensitivity of fish to dioxin-like compounds. The multiplic ity of AHR forms in fish was not predicted from knowledge of mammalian AHRs, illustrating the value of comparative studies in marine organisms, even for molecules that have been well characterized in laboratory species.
The diversity of enzymes and receptors involved in toxicity can be understood—and to a substantial extent predicted—from the evolutionary perspective gained by molecular phylogenetic analysis of genes and gene families. The duplication and diversification of genes within multigene families such as those listed in Table 1 and Table 2 is a fascinating area of basic research, which also contributes to a broader understanding of the normal function of those genes and how chemicals might interfere with that function. Recent efforts to determine the evolutionary history of steroid receptors (Escriva and others 1997; Laudet 1997), aryl hydrocarbon receptors (Hahn and others 1997), and cytochrome P450s (Morrison and others 1998; Nelson 1998, 1999) illustrate this approach.
The second type of mechanistic information needed in marine toxicology concerns the in vivo expression of the receptors and enzymes involved in toxicity, and of the genes that are under their control. Until recently, most studies have of necessity focused on measuring expression
of single genes, or at most a small number of genes. The emerging field of genomics is changing the way in which we approach such questions. Functional genomic approaches provide a way both to discover the identity of genes whose expression is altered by chemical exposure and to measure those changes rapidly and simultaneously under various conditions (DeRisi and Iyer 1999; Nuwaysir and others 1999). A functional genomic approach to measuring genome-wide changes in gene expression will be a powerful tool for the identification and quantitation of genes whose altered expression leads to toxicity. Although such studies will be restricted initially to model organisms in which extensive genome sequence data exists, this approach will become increasingly applicable and valuable with regard to marine species for which such data are not currently available.
Monitoring: Biomarkers and Bioassays
Analytical chemists have done a superb job of developing exquisitely sensitive methods for detecting contaminants and of applying those methods to generate large databases on contaminant concentrations in a variety of environmental matrices, including marine organisms. Biologists have not been nearly as successful at determining the biological significance of these concentrations.
Monitoring methods based on biological effects and their underlying mechanisms (biomarkers) can complement, and for some applications could replace, the use of analytical chemistry in monitoring the marine environment. The major advantages of such biologic, mechanism-based methods are their toxicological specificity, rapidity, and low cost. Here, “toxicological specificity” refers to the relationship between the assay response and the toxic potential (rather than simply the contaminant concentrations) of the sample being analyzed. McLachlan (1993) called this “functional toxicology.” Biological assays include in vivo biomarkers, in vivo bioassays, and in vitro bioassays.
Biomarkers are biochemical, physiologic, or other types of biological changes that indicate the presence or effects of xenobiotic compounds (CBMNRC 1987; Decaprio 1997; Huggett 1992). In addition to the commonly used biomarkers of exposure and effect, which are especially useful in biomonitoring, some biological characteristics can be used as biomarkers of susceptibility (see below). Numerous studies have shown strong relationships between in vivo biomarker responses and exposure to specific classes of marine contaminants (Huggett 1992; Stegeman and others 1992).
Biological and technological advances have facilitated the measurement of biomarkers in marine organisms, and continued improvements
are certain. Early measurements of cytochrome P450 1A (CYP1A) induction, which is widely used as a biomarker of exposure to HAH and PAH, relied on enzymatic assays (Payne 1976). The development of monoclonal antibody technology increased the specificity of assays and permitted the analysis of samples obtained from remote regions or those in which enzymatic activity might have been compromised (Stegeman and others 1986; White and others 1994). Analysis of protected species such as whales has been facilitated by recent advances in nondestructive sampling techniques, such as the ability to obtain skin biopsies, and by the identification of vascular endothelium in skin and elsewhere as a prominent site of CYP1A expression (Moore and others 1998). Such nonlethal sampling for biomarkers can be used to establish, on a global scale, the geographic variability in contaminants and their effects in marine mammals or other marine organisms.
In vivo biomarkers rely on natural exposures and responses at the level of the whole organism. Increasingly, mechanism-based bioassays employing whole animals (including transgenic animals), cultured cells, or cellular extracts are being developed and used to detect the presence of contaminants in marine samples (Hahn 2000). Examples include assays that measure receptor-binding, enzyme inhibition, or changes in gene expression. The latter assay can involve native genes such as CYP1A or reporter genes under control of defined enhancer elements that respond to the chemical and receptor of concern. The features of in vivo and in vitro bioassays and their potential for use in monitoring the marine environment have been reviewed recently (Hahn 2000).
Ultimately, a true picture of the effects of contaminants on marine systems will require long-term monitoring of these systems to evaluate changes in community structure, population genetic diversity, and other higher-level features. Evidence for such changes associated with contaminant exposure has been obtained (Guttman 1994), but the consequences of these changes are more difficult to assess. One of the more interesting phenomenon is the emergence of chemical resistance in marine animals after long-term exposure to organic or inorganic contaminants (reviewed by Hahn 1998a; Klerks and Weis 1987; Weis and Weis 1989). The mechanisms underlying such resistance in marine animals remain largely unknown.
Predicting the Impact of Marine Pollutants
Information concerning the identity and concentrations of contaminants in marine biota, along with a detailed understanding of mechanisms of toxicity and the molecular basis for species differences in sensitivity, will allow us to move toward the practical goal of predicting the
impact of specific chemicals and combinations of chemicals on marine systems. How might this occur?
One approach to achieving predictive power is through use of biomarkers of chemical effect, such as those described above. By selecting molecular markers that are closely linked to effects of concern, we can minimize the degree of uncertainty in making predictions from biomarker data.
A second approach involves using the proteins involved in causing or modulating toxicity as biomarkers of susceptibility. The development of such biomarkers is a natural outgrowth of mechanistic studies. Thus, for example, a receptor such as the AHR can serve as a biomarker of susceptibility to its ligands, in this case the dioxin-like compounds. With respect to human health, biomarkers of susceptibility are often based on interindividual variability in protein function (polymorphisms) and are linked to differences in susceptibility to disease or chemical effects (pharmacogenetics) (Nebert and others 1999; Puga and others 1997). In the context of a marine ecosystem, biomarkers of susceptibility would more likely involve species differences in protein properties that underlie differences in sensitivity. Thus, the sensitivity of marine animals might be inferred by combining information on molecular mechanisms of chemical action with data on the comparative biochemistry of proteins involved in that mechanism.
Molecular and biotechnological methods will be extremely valuable in such efforts. For example, one approach that is being used is the cloning of receptors and enzymes from marine species, followed by in vitro expression and functional analysis of the cloned proteins. Such research, for example, has revealed the catalytic properties of fish P450s, indicating that there may be subtle yet toxicologically important differences between fish and mammals in the active site of CYP1As (Doehmer and others 1999; Oleksiak and others 2000). A similar approach is being used for AHRs. Studies in inbred mice and other species have shown that the expression and properties (e.g., ligand-binding affinity) of the AHR can determine the sensitivity of animals to dioxins; this finding suggests that determining the characteristics of AHRs in marine animals could help predict their sensitivity to dioxin-like compounds (Hahn 1997). Recent studies have shown that the dioxin-binding affinity of the AHR cloned from beluga whales is unusually high, suggesting that beluga, and perhaps cetaceans generally, are among the more sensitive species to effects of these compounds (Jensen and Hahn 1999).
Finally, accurate predictions regarding effects of marine pollutants will require the additional development of mathematical models to describe the behavior of chemicals and the response of organisms to them. Existing models include those predicting the environmental fate of chemi-
cals and the exposure of organisms (Connolly 1991). As our understanding of molecular mechanisms improves, such mechanistic information will be incorporated into more realistic pharmacokinetic and pharmacodynamic models of chemical action (Limbird and Taylor 1998). Demographic models will become increasingly important in translating effects on individual organisms to the level of populations, and eventually, ecosystems (Caswell 1996). The ultimate goal will be to unite these various models to obtain integrated predictions of chemical fate and effects in marine ecosystems.
We face many challenges in our attempts to understand, monitor, and predict the impact of contaminants on marine systems. Molecular and biotechnological methods and approaches will be essential tools in the effort to meet these challenges. Some of the most compelling research needs in marine toxicology are summarized in Table 3 and in the discussion above. Marine and aquatic biologists will continue to look to the biomedical arena for many of the technological advances that will be necessary for this work. In turn, basic and applied research in marine biology, toxicology, and biotechnology will provide tools and reagents that are of great utility in biomedical research (e.g., thermostable polymerases, pharmaceuticals). Such work will also provide a broader, comparative perspective to the study of biochemical adaptation, chemical signaling, and chemical-biological interactions in biological systems.
TABLE 3. Research Needs in the Application of Molecular Biology and Biotechnology to Marine Toxicology
Molecular mechanisms underlying toxic responses and species differences in sensitivity
Molecular biomarkers
Mechanism-based bioassays
|
ACKNOWLEDGMENTS
We thank the Board on Biology, the Ocean Studies Board, and the organizers for the invitation to participate in this workshop. Preparation of this report was supported by NOAA National Sea Grant College Program Office (grant NA46RG0470, Woods Hole Oceanographic Institution [WHOI] Sea Grant project R/B-124, R/B-137 [M.E.H.], and R/P-61 [J.J.S.]), National Institutes of Health grant ES06272 (M.E.H.), US EPA grant R823890 (J.J.S.), and by the Andrew W. Mellon Foundation Endowed Fund for Innovative Research (M.E.H.). Contribution 10114 from the Woods Hole Oceanographic Institution.
REFERENCES
Abnet CC, Tanguay RL, Hahn ME, Heideman W, Peterson RE. 1999 Two forms of aryl hydrocarbon receptor type 2 in rainbow trout (Oncorhynchus mykiss): Evidence for differential expression and enhancer specificity. J Biol Chem 274:15159-15166.
Ahmed FE, ed. 1991 Seafood Safety. Washington, DC: National Academy Press.
Ballschmiter K, Froescheis O, Jarman WM, Caillet G. 1997 Contamination of the deep-sea. Mar Poll Bull 34:288-289.
Caswell H. 1996 Demography meets ecotoxicology: Untangling the population level effects of toxic substances. In: Newman MC, Jagoe CH, eds. Ecotoxicology: A Hierarchical Treatment. Boca Raton, FL: CRC/Lewis Publishers. p 255-292.
Colborn T, Saal FSV, Soto AM. 1993 Developmental effects of endocrine-disrupting chemicals in wildlife and humans. Environ Health Perspect 101:378-384.
CBMNRC [Committee on Biological Markers of the National Research Council]. 1987 Biological markers in environmental health research. Environ Health Perspect 74:3-9.
Connolly JP. 1991 Application of a food chain model to polychlorinated biphenyl contamination of the lobster and winter flounder food chains in New Bedford Harbor Environ Sci Technol 25:760-770.
Decaprio AP. 1997 Biomarkers: Coming of age for environmental health and risk assessment Environ Sci Technol 31:1837-1848.
DeRisi JL, Iyer VR. 1999 Genomics and array technology. Curr Opin Oncol 11:76-9.
Doehmer J, Buters JTM, Luch A, Soballa V, Baird WM, Morrison H, Stegeman JJ, Townsend AJ, Greenlee WF, Glatt HR, Seidel A, Jacob J, Greim H. 1999 Molecular studies on the toxifying effects by genetically engineered cytochromes P450. Drug Metab Rev 31:423-435.
Escriva H, Safi R, Hanni C, Langlois M-C, Saumitou-Laprade P, Stehelin D, Capron A, Pierce R, Laudet V. 1997 Ligand binding was acquired during evolution of nuclear receptors Proc Natl Acad Sci U S A 94:6803-6808.
Guttman SI. 1994 Population genetic structure and ecotoxicology. Environ Health Perspect 102 (Suppl 12):97-100.
Hahn, ME. 2000 Biomarkers and bioassays for detecting contamination of the marine environment. Sci Total Environ (Forthcoming).
Hahn ME. 1998a Mechanisms of innate and acquired resistance to dioxin-like compounds Rev Toxicol 2:395-443.
Hahn ME. 1998b The aryl hydrocarbon receptor: A comparative perspective. Comp Biochem Physiol 121:C23-C53.
Hahn ME. 1997 The aryl hydrocarbon (Ah) receptor: Biomarker of dioxin susceptibility? In: Proceedings of the Third Finnish Conference of Environmental Sciences, May 9-10, 1997. p 36-39.
Hahn ME, Karchner SI, Shapiro MA, Perera SA. 1997 Molecular evolution of two vertebrate aryl hydrocarbon (dioxin) receptors (AHR1 and AHR2) and the PAS family. Proc Natl Acad Sci U S A 94:13743-13748.
Hochachka PW, Somero GN. 1984 Biochemical Adaptation. Princeton, NJ: Princeton University Press. p 521.
Huggett RJ. 1992 Biomarkers for Chemical Contamination. Boca Raton, FL: CRC Press.
Jensen BA, Hahn ME. 1999 Molecular characterization of a cetacean aryl hydrocarbon receptor, a key protein involved in the toxicity of planar halogenated aromatic hydrocarbons. In: 13th Biennial Conference on the Biology of Marine Mammals, November 28 – December 3, 1999. Society for Marine Mammalogy.
Karchner SI, Powell WH, Hahn ME. 1999 Structural and Functional Characterization of Two Highly Divergent Aryl Hydrocarbon Receptors (AHR1 and AHR2) in the teleost Fundulus heteroclitus. Evidence for a novel class of ligand-binding basic helix-loop-helix Per-ARNT-Sim (bHLH-PAS) factors. J Biol Chem 274:33814-33824.
Klerks PL, Weis JS. 1987 Genetic adaptation to heavy metals in aquatic organisms: a review Environ Poll 45:173-205.
Laudet V. 1997 Evolution of the nuclear receptor superfamily: Early diversification from an ancestral orphan receptor. J Mol Endocrinol 19:207-226.
LeBlanc GA. 1994 Assessing deleterious ecosystem-level effects of environmental pollutants as a means of avoiding evolutionary consequences. Environ Health Perspect 102:266-267.
Limbird LE, Taylor P. 1998 Endocrine disruptors signal the need for receptor models and mechanisms to inform policy. Cell 93:157-163.
Livingstone DR, Stegeman JJ. 1998 Forms and functions of Cytochrome P450. Comp Biochem Physiol 121C:1-412.
May RM. 1988 How many species are there on earth? Science 241:1441-1449.
McLachlan JA. 1993 Functional Toxicology: A new approach to detect biologically active xenobiotics. Environ Health Perspect 101:386-387.
Moore MJ, Miller CA, Weisbrod AV, Shea D, Hamilton PK, Kraus SD, Rowntree VJ, Patenaude N, Stegeman JJ. 1998 Cytochrome P450 1A and chemical contaminants in dermal biopsies of northern and southern right whales. In: Workshop on the Comprehensive Assessment of Right Whales, Cape Town, South Africa, March 19-25, 1998. International Whaling Commission.
Morrison HG, Weil EJ, Karchner SI, Sogin ML, Stegeman JJ. 1998 Molecular cloning of CYP1A from the estuarine fish Fundulus heteroclitus and phylogenetic analysis of CYP1A genes: Update with new sequences Comp Biochem Physiol 121:C231-C240.
Muir DCG, Norstrom RJ, Simon M. 1988 Organochlorine contaminants in arctic food chains: Accumulation of specific polychlorinated biphenyls and chlordane-related compounds Environ Sci Technol 22:1071-1079.
Nebert DW, Ingelman-Sundberg M, Daly AK. 1999 Genetic epidemiology of environmental toxicity and cancer susceptibility: Human allelic polymorphisms in drug-metabolizing enzyme genes, their functional importance, and nomenclature issues. Drug Metab Rev 31:467-87.
Nelson DR. 1998 Metazoan cytochrome P450 evolution. Comp Biochem Physiol 121:C15-C22.
Nelson DR. 1999 Cytochrome P450 and the individuality of species. Arch Biochem Biophys 369: 1-10.
NRC [National Research Council]. 1999 Hormonally Active Agents in the Environment. Washington, DC: National Academy Press.
Nuwaysir EF, Bittner M, Trent J, Barrett JC, Afshari CA. 1999 Microarrays and toxicology: The advent of toxicogenomics. Mol Carcinog 24:153-9.
Oleksiak MF, Wu S, Parker C, Karchner SI, Stegeman JJ, Zeldin DC. 2000 Identification, functional characterization and regulation of a new cytochrome P450 subfamily, the CYP2Ns. J Biol Chem 275:2312-2321.
Payne JF. 1976 Field evaluation of benzopyrene hydroxylase induction as a monitor for marine pollution. Science 191:945-946.
Pimm SL, Russell GJ, Gittleman JL, Brooks TM. 1995 The future of biodiversity. Science 269:347-350.
Prosser CL. 1986 Adaptational Biology. Molecules to Organisms. New York: John Wiley & Sons. 766 p.
Puga A, Nebert DW, McKinnon RA, Menon AG. 1997 Genetic polymorphisms in human drug-metabolizing enzymes: Potential uses of reverse genetics to identify genes of toxicological relevance Crit Rev Toxicol 27:199-222.
Schmidt JV, Bradfield CA. 1996 Ah receptor signaling pathways. Annu Rev Cell Devel Biol 12:55-89.
Stegeman JJ, Brouwer M, DiGiulio RT, Forlin L, Fowler BM, Sanders BM, Van Veld P. 1992 Molecular responses to environmental contamination: Enzyme and protein systems as indicators of contaminant exposure and effect. In: Huggett RJ, editor. Biomarkers for Chemical Contaminants. Boca Raton, FL: CRC Press. p 237-339.
Stegeman JJ, Hahn ME. 1994 Biochemistry and molecular biology of monooxygenases: Current perspectives on forms, functions, and regulation of cytochrome P450 in aquatic species. In: Malins DC, Ostrander GK, eds. Aquatic Toxicology: Molecular, Biochemical and Cellular Perspectives Boca Raton, FL: CRC/Lewis. p 87-206.
Stegeman JJ, Kloepper-Sams PJ, Farrington JW. 1986 Monooxygenase induction and chlorobiphenyls in the deep-sea fish Coryphaenoides armatus. Science 231:287-1289.
Weaver G. 1984 PCB contamination in and around New Bedford, MA. Environ Sci Technol 18:22A-27A.
Weis JS and Weis P. 1989 Tolerance and stress in a polluted environment. Bioscience 39:89-95.
White RD, Hahn ME, Lockhart WL, Stegeman JJ. 1994 Catalytic and immunochemical characterization of hepatic microsomal cytochromes P450 in beluga whales (Delphinapterus leucas). Toxicol Appl Pharmacol 126:45-57.