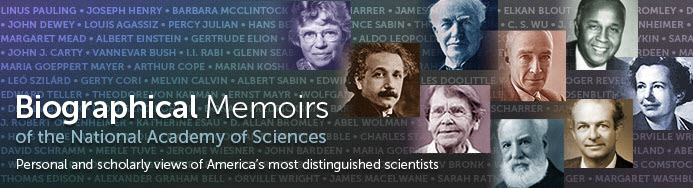
NEW MEMOIRS
- Jonathan J. Cole (1953-2023)
by Gene E. Likens, Stephen R. Carpenter, and Michael L. Pace (Environmental Sciences and Ecology)
- Arthur H. Lachenbruch (1925-2021)
by Barbara Lachenbruch (Geology)
- Arthur Dodd Code (1923-2009)
by David H. DeVorkin and Jordan D. Marché II (Astronomy)
HIGHLIGHTS
Published since 1877, Biographical Memoirs provide the life histories and selected bibliographies of deceased National Academy of Sciences members. Colleagues familiar with the subject's work write these memoirs and as such, the series provides a biographical history of science in America.
The Online Collection includes approximately 1,900 memoirs, including those of famed naturalist Louis Agassiz; Joseph Henry, the first secretary of the Smithsonian Institution; Thomas Edison; Alexander Graham Bell; noted anthropologist Margaret Mead; and psychologist and philosopher John Dewey.